J. Northw. Atl. Fish. Sci., Vol. 55: 31–58
Pierre Pepin1*
, Frances K. Mowbray1, Hannah M. Murphy1
, Dominique Robert2
, Paul V.R. Snelgrove3
1Fisheries and Oceans Canada, Northwest Atlantic Fisheries Centre,
St. John’s, NL Canada A1C 5X1
2Université du Québec à Rimouski, Institut des sciences de la mer de Rimouski,
Rimouski, QC Canada G5L 3A1
3Memorial University of Newfoundland, Ocean Sciences Centre,
St. John’s, NL Canada A1C 5S7
*Corresponding author: pierre.pepin.nl@gmail.com
Pepin, P., Mowbray, F.K., Murphy, H.M., Robert, D., and Snelgrove, P.V.R. 2024. Seasonal succession of the larval fish community from coastal areas of eastern Newfoundland, Canada. J. Northw. Atl. Fish. Sci., 55: 31–58. https://doi.org/10.2960/J.v55.m749
Abstract
We present a synthesis of all sampling programs aimed at collection of ichthyoplankton conducted in coastal waters of eastern Newfoundland during the period 1982–2016, describing seasonal patterns in the succession of 22 taxa in terms of diversity, probability of occurrence, and overall abundance. Additionally, we assess whether changes in these patterns occurred prior to and after the collapse of major commercial fish stocks and accompanying shifts in ecosystem structure. Despite differences in relative species composition and community structure among bays, we identified clear and repeatable patterns in the seasonal cycle of species succession from Placentia Bay, on the south coast, to White Bay on the northeastern coast of the island. Some of the differences among bays reflected latitudinal patterns in the timing of the seasonal environmental cycle, with increasing delays toward northerly bays. Relatively few species generally dominated the larval fish community, although dominance varied seasonally, particularly once emergence of capelin commenced in July and August. Abundance of larval fish reflected the changes in abundance of adult stocks of commercial species, with strong declines in density following stock collapses, but larval decreases extended to non-commercial coastal taxa potentially removed as bycatch or through impacts of changes in ocean conditions that contributed to the decline of major ecosystem stocks (e.g., Atlantic cod, capelin, American plaice). Although differences in timing and extent of ichthyoplankton collections confounded our data, the general consistency of our observations with other sources of information (e.g., research vessel trawl surveys) highlights the value of such collections to monitoring of areas poorly represented by other types of surveys. Knowledge of changes in larval fish community structure in coastal areas can set a foundation to understand better the potential interaction between anthropogenic and climate impacts on the ecosystem state in coastal areas.
Keywords: biodiversity, community structure, ecosystem state, ichthyoplankton, reproduction, spawning cycle
PDF, Supplementary Materials
Download Citation Data

Citation to clipboard
Reference management software (Endnote, Mendeley, RefWords, Zotero & most other reference management software)

LaTex, BibDesk & other specific software
Introduction
Atlantic cod (Gadus morhua) populations on the Newfoundland and Labrador continental shelves collapsed in the late 1980s and early 1990s as a result of overfishing and changes in ocean conditions (Lilly et al., 2013) concurrent with major changes in the broader marine ecosystem (Gomes et al., 1995; Pedersen et al., 2017). The precipitous decline in the northern cod stock (e.g., Myers et al., 1997), paralleled the abrupt decline in abundance of capelin (Mallotus villosus), a keystone forage species (Buren et al., 2014; Buren et al., 2019), along with dramatic changes in other commercial and non-commercial species (Pedersen et al., 2017). Other studies provide evidence of changes in life history characteristics and production potential of several stocks (Stares et al., 2007; Morgan Tripp et al., 2024). Despite evidence of a partial return of regional ecosystems to their historical structure in the mid-2010s, most stocks remain depleted (Koen-Alonso and Cuff, 2018) and substantial changes in community structure persist (Aune et al., 2024). Although many factors contributed to this regional crisis, Harris (1990) noted that achieving a basic understanding of the Newfoundland shelf ecosystem, beyond data on adult fish of commercial stocks, represented an important source of information that could reduce future risks in the provision of management advice.
Ichthyoplankton surveys provide a means to understand the biology and ecology of fish populations, an essential component to forecasting the effects of changes in climate and ecosystem state on regional productivity and resilience (Koslow and Wright, 2016). Extensive programs studying early life stages of fish have focussed on understanding the drivers of change in stock productivity. However, multidisciplinary population level research programs are often limited in duration (e.g., GLOBEC – a study of Global Ocean Ecosystem Dynamics (Lehodey et al., 2006; Batchelder and Kim, 2008; Voss et al., 2012; Fogarty et al., 2013)) and thus base their inferences and conclusions on only a few cohorts of a given taxon. Such programs also seldom consider aspects of ichthyoplankton community structure. There are relatively few long-term observation programs focus on larval fish dynamics (e.g., North Sea (Simmonds, 2009), California Current (Gallo et al., 2019), Japan (Oozeki, 2018), Bering Sea (Goldstein et al., 2019)). Nevertheless, collections of fish eggs and larvae have provided fishery-independent estimators of spawning stock abundance (e.g., Fissel et al., 2011; Dickey-Collas et al., 2012; Somarakis et al., 2012), predictors of year-class strength (e.g., Auth and Brodeur, 2013; Islam et al., 2015), and changes in climate driven community composition (e.g., Boeing and Duffy-Anderson, 2008; Morson et al., 2019; Nielsen et al., 2021). Seasonal sampling programs can also provide insights into the timing of reproductive activity, filling a particularly useful niche where scientific research surveys do not effectively collect data on all relevant species. Ichthyoplankton surveys can also identify ecosystem elements or factors that influence early life survival, a critical aspect of recruitment variability (Houde, 2008; Pepin, 2016), as well as provide insights into shifts in community composition or the introduction of new taxa to a region (e.g., Peterson et al., 2022). Tracking of larval fish cohorts through time has informed studies on patterns of variation in growth rates (Hare and Cowen, 1997; Robert et al., 2007; May et al., 2020) and the potential impact of predators (Robert et al., 2010; Takasuka et al., 2017; de Queiroz et al., 2024). Because of high vulnerability of fish larvae to changes in feeding conditions and sources of loss (Houde, 1987; Beyer, 1989), ichthyoplankton studies provide a strong foundation to investigate how changes in ecosystem conditions and productivity can affect natural resource management (e.g., Möllmann et al., 2008; Siddon et al., 2011; Marshall et al., 2019). In short, basic knowledge on the occurrence, abundance, and distribution of early life history stages across a broad range of taxa offers critical information to understand how underlying processes may affect the stability of food webs and fish populations.
Coastal environments represent areas of high biodiversity and productivity (DFO, 2019) but the focus on management of commercially important and broadly distributed fish and invertebrate stocks in nations with highly developed industrial fisheries often limits knowledge of ecosystem features and dynamics. These regions of high productivity are typically vulnerable to changes in atmospheric and climate forcing (e.g., Evans and Pepin, 1989; Tian et al., 2003). Complex habitat structure and diversity characterize coastal regions (e.g., Proudfoot et al., 2020; Amani et al., 2022), often enhancing reproductive opportunities (e.g., nesting sites, algal cover, complex geomorphology) and providing diverse nursery sites for a broad range of taxa (e.g., Ings et al., 2008; Renkawitz et al., 2011; Dalley et al., 2017). Wind-driven changes in circulation and water mass distributions in coastal environments can affect the dynamics of ichthyoplankton by altering retention of eggs and larvae in suitable habitats, or shifting their overlap with potential prey and predators (Leggett et al., 1984; Grant and Brown, 1998; Snelgrove et al., 2008; Stanley et al., 2013).
The more than 17 500 km of coastlines in Newfoundland and Labrador encompass many physically dynamic bays and fjords, for which numerous studies have investigated the response of ichthyoplankton species to fluctuations in ocean currents and productivity (Laprise and Pepin, 1995; Pepin et al., 1995; Bradbury et al., 2003; Snelgrove et al., 2008; Stanley et al., 2013). A subarctic Atlantic plankton community characterizes the Labrador Northeast-Newfoundland shelf ecosystem (Davis, 1982; Davis, 1986; Pepin et al., 2011); however, most reports of seasonal variation in the occurrence, abundance, diversity and dynamics of early life stages of fish have focused on single species from a single bay (e.g., Leggett et al., 1984; Bradbury et al., 2003; Pepin et al., 2003; Bradbury et al., 2008; Murphy et al., 2018; Wilson et al., 2018). Effective use of information from coastal areas to inform and supplement other approaches aimed at monitoring of ecosystem state remains a challenge. Our study aims to synthesize data from multiple ichthyoplankton surveys conducted in coastal Newfoundland bays in recent decades to:
We predict similarities in species composition among bays but also a seasonal progression in community structure that follows a latitudinal progression reflective of differences in the local environmental cycle. Differences in species ranges of adult fish may also affect overlap in taxonomic composition among sites. We base this synthesis on the amalgamation of multiple ichthyoplankton collections aimed at providing advice concerning the management of fish stocks and cycles of abundance, investigations into processes affecting the growth and mortality of larval fish, and studies into the dispersal and retention of commercially important species. We collected data from sampling throughout eastern Newfoundland, from April through October, between 1982 and 2016, acknowledging that variation in year, month, and bay confounded sampling activities throughout this period. Collections ranged from White Bay on the northeast coast of Newfoundland to Placentia Bay on the south coast (Fig. 1). Although suboptimal, a synthesis based on multiple sources of data generated for a wide range of purposes nonetheless provides a critical first step to understanding variation in the dynamics of coastal ichthyoplankton in the absence of comprehensive knowledge of the general patterns of larval fish occurrence in a broad geographic region. Such an approach provides a foundation to develop more comprehensive assessments of the reproductive cycles of fishes in the region and to identify critical information gaps.
Fig. 1
Oceanographic setting
The flow of the inner branch of the Labrador current strongly influences coastal areas of the Newfoundland continental shelf and Grand Banks (Loder et al., 1998). White, Notre-Dame, Bonavista, Trinity, and Conception Bays face in a northeastern direction, in contrast to southwestern facing Placentia Bay (Fig. 1), resulting in differential effects of wind forcing. In the Newfoundland region, southwesterly winds prevail and induce upwelling in northeast facing bays, whereas northeasterly winds likely suppress upwelling and intensify surface heating (Yao, 1986; deYoung et al., 1993a; deYoung et al., 1993b; deYoung and Sanderson, 1995; Davidson et al., 2001; Ma et al., 2012). Average currents are generally weak and highly variable, with largely meagre tides (~2–3 cm·s-1).Local winds drive surface circulation on short time scales (hours to days) but density-driven exchanges with shelf waters and the inshore branch of the Labrador current drive circulation on longer time scales (weeks to months). Storms can generate Kelvin waves trapped within about 5 km of either coast with associated jet-like currents (deYoung et al., 1993a; deYoung et al., 1993b). Salinity strongly influences stratification, and summer mixed layer depth ranges from 10–40 m, with an internal Rossby radius of ~5–8 km. Sub-zero temperatures in the cold intermediate layer form locally in winter on the Newfoundland and Labrador shelf, typically below 50 m and extending to depths exceeding 150 m near the coast (Petrie et al., 1988). Considerable large-scale coherence characterizes seasonal and interannual variation in oceanographic conditions and their response to fluctuations in atmospheric forcing (DFO, 2023).
The seasonal temperature and salinity cycles, at a high frequency sampling site (Station 27– 47.55°N, 52.59°W) on the east coast of Newfoundland (Petrie et al., 1991; Petrie et al., 1992), demonstrate notable fluctuations in surface waters and more limited variation with increasing depth (Supplementary Fig. 1). Stratification starts in March/April through a combination of changes in temperature and salinity. Surface temperature reaches a maximum in August/September, and minimum in January/February. Notable freshening of the surface layer occurs in May and reaches a maximum in September. Mesozooplankton abundance at Station 27 is lowest in May and increases progressively until September (Supplementary Fig. S2). There are no reliable monitoring data concerning the seasonal cycle of copepod nauplii, the principle prey of fish larvae, other than that reported by Pepin (2023). Copepods dominated non-copepod taxa throughout the year, with highest proportional abundance of non-copepods between May and October, and lowest in winter. Copepod taxa most important in the diet of larval fish (Oithona spp., Pseudocalanus spp., Calanus finmarchicus, and Temora longicornis) generally comprise more than 80% of the abundance of copepodite stages. Strong seasonal variability characterized taxonomic composition of non-copepods.
Environmental conditions
Fig. 2
Numerous studies have documented the environmental history of the Newfoundland Shelf ecosystem. Most recently, the Newfoundland and Labrador Climate Index (NLCI – Cyr and Galbraith (2021)), composed of 10 variables that quantify atmospheric, ice, and oceanographic variables summarizes broad-scale regional conditions and demonstrates cyclic patterns of variation over time (Fig. 2). In general, the patterns of change in the NLCI demonstrate that increased variability, quantified by the 11-year running average of the standard deviation in NLCI, occurs every 7–9 years, with the exception of the most recent period (2005–present) when overall variability has remained high. From 1951 to 1970 physical conditions fluctuated around an average state, with some elements (e.g., air temperature, salinity) generally below the 1991–2020 average, in contrast to other elements of the index that were generally above normal. From 1970 to 1980, the NLCI abruptly declined to below normal levels, but most elements of the index gradually increased to above normal by 1981. After this improvement, environmental conditions declined rapidly to below normal for an extensive period (1982–1996), although with pronounced fluctuations during this period in some index elements. Since 1998, the NLCI has generally remained above normal, although with considerable short-term variability (~5 year periodicity) that likely results from climate change.
Extensive analyses document the collapse of the northern cod stock in the late 1980s and early 1990s (e.g., Myers et al., 1997), but the combined effects of over-exploitation and environmental change also resulted in a decline and major shift in the structure and distribution of the remainder of the groundfish community (Gomes et al., 1995; Pedersen et al., 2017). Capelin, a keystone forage species of the Newfoundland ecosystem, also collapsed in the early 1990s, coincident with the decline in demersal fish biomass (Buren et al., 2019). These changes were associated with a shift in overall community structure that resulted in increased overall biomass of shellfish in the 1990s, mainly in the form of snow crab (Chionoecetes opilio) and northern shrimp (Pandalus borealis), which peaked in the mid-2000s and have since declined (Aune et al., 2024). Similar shifts from groundfish to shellfish have been reported in other geographic regions (Worm and Myers, 2003). Coincident with the decline in invertebrate stocks, a modest recovery of groundfish stocks occurred, but which has yet to reach pre-collapse levels of biomass, on either the Newfoundland Shelf or the Grand Banks (NAFO, 2023).
Ichthyoplankton surveys
The ichthyoplankton data encompass surveys of Trinity and Fortune Bay (1982–1986) aimed at monitoring herring populations, process studies conducted in Conception, Trinity, or Bonavista Bays (1985–1986, 1990–2013), seasonal surveys of Placentia Bay (1997–1999), and monitoring surveys of capelin in Trinity Bay (2002–2016). Monitoring surveys used 60-cm bongo nets with 333-µm mesh towed obliquely from the surface to 200 m. Most process studies used a 4 m2 Tucker trawl with variable mesh sizes (Pepin and Shears, 1997), towed obliquely from the surface to 50 m, and occasional use of 60 cm bongo nets, as did the surveys of Placentia Bay. One process study in Conception Bay 1985–1986 used a 0.75 m ring net towed horizontally at a depth of 7 m. Vessel speed varied between 1.5 and 2.5 m·s-1. All nets were equipped with flowmeters to estimate the distance towed. Abundance of each species at each station was calculated as N=CD/AL where N is the number of larvae·m-2, C is the number of larvae collected (n), D is the maximum sample depth (m), L is the distance towed (m), and A is the area of the net opening (m2). Tow depth differences among surveys required application of area-based estimates of density.
The combined surveys collected a total of 5 022 samples throughout eastern Newfoundland, from April through October, between 1982 and 2016, and encompassed 98 separate research missions. Some process-oriented missions involved up to 3 surveys of the bay under study, and our analyses considered each survey separately. Surveys of Placentia Bay (April to August), Conception Bay (April to September) and Trinity Bay (May to October) provided relatively complete coverage of the seasonal cycle of larval fish occurrence, in contrast to limited seasonal coverage of Notre Dame and White Bays (July, August, and September), and Fortune and Bonavista Bays (July only). Because of limited temporal collections, we grouped data from Fortune and Bonavista Bays with information from the adjacent Placentia and Trinity Bays, respectively. Only data from Conception and Trinity Bays provided community structure prior to (1980s) and after (1990–2016) the major shift in the Newfoundland coastal ecosystem. The number of samples per mission ranged from 5 to 205, with a median of 34. We note the unbalanced sample collection across years and months, with sample collections heavily weighted toward the 1980s (56% of samples collected between 1982–1986), and an emphasis on warmer/summer months when we anticipated highest larval abundances (74% of samples collected in June, July, and August (Fig. 3)). We acknowledge large gaps in sample collection, both in time and geography, with very few collections occurring in early spring and fall (Fig. 3). This bias primarily reflects the opportunistic nature of sample collections, vessel availability, and project objectives. Sample collection also heavily favoured two locations, with 55% of samples collected in Trinity Bay and 30% collected in Conception Bay (Fig. 3).
Fig. 3
All samples were preserved in 4–5% buffered formaldehyde, with the exception of projects aimed at otolith-based estimation of growth rates that used 95% ethanol. Ichthyoplankton were sorted from samples and identified to the lowest taxonomic level possible (Fahay, 1983; Fahay, 1992a; Fahay, 1992b, and references therein). For this analysis we identified 22 taxa (Table 1), representing 15 families, and with 16 taxa reliably identified to species level. We combined six taxa to genus level because of variations in technical and taxonomic expertise among the different surveys and studies that resulted in inconsistent levels of identification. Ammodytes sp. and Pholis sp. likely each consisted of a single species (Ammodytes dubius and Pholis gunnellus, respectively), based on Scott and Scott (1988). Specimens of Lumpenus spp. identified to species consisted principally of Leptoclinus macculatus (formerly accepted as Lumpenus macculatus) with a few specimens of Lumpenus lampretaeformis. Liparis atlanticus likely dominated Liparis spp. (Pepin, unpublished data), with Liparis gibbus as the second most frequently identified species, along with a few specimens identified as Liparis inquilinus and Liparis liparis. Larvae of Myoxocephalus spp. that could be identified to species included Myoxocephalus scorpius, Myoxocephalus scorpioides, Myoxocephalus octodecemspinosus and Myoxocephalus aenaeus, but the majority of specimens were not identified to species level. Sebastes spp. could not be identified to species level but likely consisted of Sebastes norvegicus (formerly Sebastes marinus) and/or Sebastes fasciatus on the east coast, although Sebastes mentella also occurs on the south coast of Newfoundland, in proximity to Placentia Bay. Infrequent taxa, identified in fewer than 90 samples included: Liopsetta putnami (Pleuronectidae), Pollachius virens (Gadidae), Anarhichas lupus (Anarhichadidae), Gymnocanthus tricuspis (Cottidae), Icelus sp. (Cottidae), Triglops sp. (Cottidae), Hemitripterus americanus (Hemitripteridae), Urophycis sp. (Phycidae), Hippoglossus hippoglossus (Pleuronectidae), and Gaidropsarus sp. (Gaidropsaridae), in descending order of relative occurrence. When frequent and infrequent taxa from our collections are considered, Pleuronectidae was the most specious family (6), followed by Cottidae (4), Stichaeidae (3), Gadidae (3), Agonidae (2), with all other families represented with only one consistently identified species. However, Cottidae, Stichaeidae, Liparidae and Sebastidae likely consisted of 7, 4, 4, and 3 species, respectively, but identification to species were inconsistent or not possible. Egg type and diameter were collated from Scott and Scott (1988), Fahay (1992a) and Fahay (1992b). Taxonomic names were verified from the World Register of Marine Species (https://www.marinespecies.org/).
Table 1
Analyses
The first part of the analytical approach of this study involved the use of multivariate methods aimed at synthesizing and describing general seasonal community structure, and evaluating patterns of change in diversity among the different geographic areas around Newfoundland. These analyses address the first two objectives described in the introduction. The second part of the analyses aimed to describe the seasonal cycle of individual species, the variation in length of larvae (minimum and maximum), and contrast the overall abundance prior and after the collapse of major fish stocks, and the substantial changes in ecosystem state that occurred at the same time.
Ichthyoplankton community
Analytical design of this study was developed from explorations of observations from the various collections and data series. To describe overall seasonal patterns of larval production, the first steps focussed on overall abundance and diversity, along with comparison of species richness and Shannon’s diversity index among bays. We followed this analysis with a description of the average seasonal succession of fish taxa in Placentia, Conception, Trinity, and the combined data from Notre Dame and White Bays (hereafter referenced as the northern bays). This description of the seasonal abundance and probability of occurrence of each taxon synthesizes patterns of reproduction of dominant fish in coastal Newfoundland. Finally, a multivariate comparison of community structure among all bays and prior to and following the major change in the Newfoundland coastal ecosystem provided a means to quantify and contrast species composition.
Abundance, richness and diversity
We provide a general description of the seasonal cycle in overall ichthyoplankton abundance, and variation in density within surveys and among sampling stations, based on the sum of all fish larvae within each sample.
We estimated alpha diversity for each sample as species richness (N0 = q, where q is the number of taxa) based solely on taxa presence, giving rare and abundant taxa equal importance. For each sample we estimated Shannon’s entropy (, where pi is the proportion of each species present in each sample), with the maximum value dependent on overall species richness of the sample (ln q). Shannon’s entropy diminishes the relative weight of rare species.
To assess our ability to evaluate beta diversity, we estimated the pattern of variation in species composition among sites in the geographic area of separate surveys within research missions, when applicable, based on the approach developed by Baselga (2012). This approach separates the elements of nestedness, when the biotas of sites with smaller numbers of species represent subsets of the biotas at richer sites, from spatial turnover, which involves the replacement of some species by others from site to site. Jaccard dissimilarity (βJAC) is the sum of the turnover component of Jaccard dissimilarity (βJTU) and nestedness-resultant component of Jaccard dissimilarity (βJNE). Estimates of dissimilarity components were obtained using R package betapart (Baselga et al., 2023) using R 4.3.1 (R Core Team, 2023). Baselga (2010) noted high sensitivity to sample size in comparison of beta diversity components among sites, a real concern for our study because of substantial variation in numbers of sites sampled among the various surveys collated for this synthesis. Baselga (2010) used randomization to contrast sites with different numbers of observations, but such an approach would place severe limitations on the inferences we could draw in comparing surveys with fewer sites relative to more comprehensive collection programs. Instead, we used all of our surveys and estimated the dependency in the three dissimilarity metrics on the number of samples contributing to each survey. Based on a priori data exploration, we fitted estimates of both βJAC and βJTU to a three parameter exponential rise to a maximum (βi=βi,0+ai1-e-biN, where βi is either βJAC or βJTU, N is sample size, and ai and bi are estimated parameters, which we report as average ± standard error), whereas we fit a three parameter exponential decay to estimates of βJNE (βi=βi,0+aie-biN). Understanding the sample size dependency in estimates of dissimilarity provides insight into survey requirements to evaluate effectively how to quantify beta diversity in coastal regions, and assess potential factors and processes driving the larval fish community. An analysis of variance evaluated whether the residuals from these relationships differed significantly among bays and ecosystem states.
Seasonal occurrence cycle
We based our description of the seasonal cycle in the occurrence and production of larval fish across all bays on the presence of each fish taxon in all the samples from all research missions over 10-day time intervals. We then estimated the probability of occurrence as the sum of samples in which each taxon was present relative to the total number of samples collected during each time interval. Assessment of patterns in the seasonal production of larval fish, a metric of reproductive activity, used group-average clustering based on square-root transformation of the probability of occurrence among all samples from all bays. These comparisons used PRIMER's CLUSTER algorithm combined with an evaluation of the similarity profile test (SIMPROF) (Clarke and Gorley, 2006).
Species composition and community structure
We developed a general description of the species composition among bays from the relative species composition based on the arithmetic average density of fish larvae from all samples, including zero values, in each month for each bay with seasonal coverage. We defined the relative contribution of each taxon qualitatively as minor for species with a maximum relative contribution to species composition of <3%, moderate for species with maximum relative contribution of 3–10%, major for species with maximum relative contribution of 10–30%, and dominant for those with a maximum contribution >30%.
To evaluate the seasonal cycle in larval fish composition, we used a principal coordinate analysis (PCoA) of community structure, which provides a relative comparison of different bays and ecosystem states in multivariate space. A PERMANOVA (permutational multivariate analysis of variance) comparison based on location (bay), month, their interaction, and period of ecosystem state (pre-collapse [1982–1986] and post-collapse [1990–2016]), evaluated whether either of these factors had a statistically significant influence on similarity and dissimilarity in species composition by testing for pairwise differences among groups. An analysis of similarity (ANOSIM) evaluated the findings of the PERMANOVA, but based on a non-parametric assessment of the distances among samples within groups relative to distances among groups. This approach thus provided a sense of scale of the findings of the parametric analysis. A two-way analysis of similarity/dissimilarity percentages (SIMPER) identified which species had greater influence in differentiating among bays and months using pairwise comparisons. The PCoA, PERMANOVA, and ANOSIM used a resemblance matrix of community composition abundance data of all the samples based on the Hellinger distance (Legendre and Gallager, 2001). SIMPER two-way (bay, month) analyses used both Euclidean distance of log-transformed abundance data and a Bray-Curtis similarity matrix based on presence/absence transformation. These multivariate analyses explored the composition of patterns of variation in the larval fish community in coastal Newfoundland. All multivariate analyses were conducted using PRIMER v 6.1.13 (Clarke and Gorley, 2006) and PERMANOVA+ v 1.0.3 (Anderson et al., 2008).
Species specific cycles
For each taxon, we report the data and narrative describing seasonal cycles in probability of occurrence, average density, and minimum and maximum length in relation to day of year in the supplementary material. Separation of the data from Conception and Trinity Bays into pre- and post-collapse periods provided a comparison between ecosystem states. We based the seasonal cycle in the occurrence and production of each taxon of larval fish on the presence of each fish taxon in all the samples over 10 day time intervals for each research mission and bay. To estimate the probability of occurrence, we summed the number of samples in which each taxon was present relative to the total number of samples collected during each interval, bay and research mission, and reported it in relation to the average day of year of each of those sample collections. We reported patterns in the seasonal production of larval fish for each species, bay and research mission, a metric of reproductive activity, as the arithmetic average density (larvae·m-2) based on all non-zero observations from the aggregation of data subsets. We established a cutoff of ~ 1 larva·100 m-2 for most reporting to provide scope in the graphics presented in the supplementary material. Because data on larval length were reported unevenly among the various data sources, we used only the data from Conception Bay to estimate the minimum and maximum length of larvae present, estimated for each day of the year across all research missions in that Bay. Length observations provided an indicator of spawning or reproduction when small individuals were present, as well as a metric of larval development, in relation to day of year.
To contextualize our new knowledge of temporal shifts in timing of reproduction or spawning activity achieved from our analyses, we contrasted our observations with information from syntheses for the northwest Atlantic (Scott and Scott, 1988; Fahay, 1992a; Fahay, 1992b). To ensure that we included the latest information available, we conducted a search of the Web of Science using the search terms TS = taxon name AND PY = 1986–2024 AND (TS = larva* OR TS = egg*).
We based the order of presentation of taxa on the outcome of the cluster analysis of the seasonal cycle in the occurrence of larval fish, and estimated the average day of occurrence as the weighted probability of occurrence on the midpoint for each 10 day interval (i), starting from day 90, across all bays (, for pi > ٠).
Results
Ichthyoplankton community
Abundance, richness and diversity
Seasonal change in total average larval fish density in all coastal bays varied by 3.2, 2.8 and 1.5 orders of magnitude in Placentia, Conception, and Trinity Bays, respectively (Fig. 4). Average within-survey total density varied by 1.78 orders of magnitude (range: 0.4 – 4.0), demonstrating a high degree of spatial heterogeneity in the abundance of fish larvae within bays.
Fig. 4
Low species richness in May, June, and September contrasted with highest values in July and August (Fig. 5), and richness was generally greater in Conception and Placentia Bays relative to Trinity and northern Bays. A maximum of 13 taxa occurred in a given sample from single surveys, although total richness among samples from that location was generally greater. There were 766 samples with only one taxon present. Despite generally similar Shannon’s entropy among bays, a greater range of values characterized Conception and Trinity Bays relative to other locations, indicative of a more even distribution in relative abundance within samples. The limited number of research missions from Notre Dame and White Bays limited our capacity to derive inferences in the comparison with other bays. The distribution of Shannon’s entropy only achieved near maximum values for richness levels of 5 species or fewer (Supplementary Fig. S3). Median entropy increased from 0.48 at richness of 2 to 0.98 at richness of 8 taxa, after which it declined to a value of ~ 0.5 at the highest richness levels. Median entropy was 69% of the expected maximum at a richness of 2 and progressively declined to ~20% of the expected maximum at the highest richness levels, indicative of increasing relative dominance of one or a few taxa in each sample as overall richness increased.
Fig. 5
Variation in Jaccard dissimilarity (βJAC), as well as elements of nestedness and spatial turnover, depended strongly on the number of sampling sites among surveys (Fig. 6). All non-linear relationships were statistically highly significant (p < 0.001). Both βJAC and βJTU reached an asymptote (0.97 and 0.94, respectively) but their intercepts (β0,JAC = 0.37 (± 0.054), β0,JTU = 0.21 (± 0.082), respectively) and rates of increase (βJAC = 0.093 (± 0.0093), bJTU = 0.058 (± 0.0098)) differed significantly. The exponential decline in nestedness (βJNE) to a low of 0.03 at a large sample size was highly significant (p < 0.001). The inflexion point in Jaccard dissimilarity (βJAC) occurred at a sample size of ~25. However, the contribution of nestedness and spatial turnover varied greatly until sample size reached ~50 stations, with particularly high variability at a sample size of 25 stations or fewer. These patterns indicate that the processes of larval production (e.g., hatching, emergence from demersal sites), physical mixing, and transport reach an equilibrium in their contribution to beta diversity patterns only once the entire bay has been sampled comprehensively. The residuals of Jaccard dissimilarity (p < 0.05) and nestedness (p < 0.05) differed significantly among bays, but spatial turnover did not (p > 0.1). However, only one bay contributed to the significant results, pre-collapse Conception Bay for Jaccard dissimilarity, and post-collapse Trinity Bay for nestedness, with extreme outliers contributing heavily to these outcomes, suggesting only moderate differences among coastal areas.
Fig. 6
Seasonal occurrence cycle
Cluster analysis of the seasonal cycle in larval fish occurrence across all bays revealed groupings reflective of the timing of larval production and period during which larvae of each taxon occurred in the plankton. Gunnel (Pholis sp.), alligatorfish (Aspidophoroides monopterygius), sculpin (Myoxocephalus spp.), sand lance (Ammodytes sp.), and blenny (Lumpenus spp.) (cluster b – Fig. 7, Supplementary Figure S4) larvae appeared first in the spring, with peak occurrence in late March/early April, likely indicative of winter spawning (Supplementary Figs. S5–S9). Sand lance and blenny were seasonally dominant taxa in Placentia and Conception Bays, respectively, whereas sculpin and gunnel were moderate contributors to species composition, and alligatorfish were minor contributors to the larval community (Fig. 8). Clustering linked the occurrence of Arctic shanny (Stichaeus punctatus) (cluster a) (Supplementary Fig. S10) with that of the previous group (cluster b) with a similarity of 62.5%, but Arctic shanny first appeared in April and peaked in late May, at which times they were dominant contributors to community structure (Fig. 8). The next group (cluster c), with 45% similarity with the previous two clusters, consisted of Arctic cod (Boreogadus saida) and Atlantic poacher (Leptagonus decagonus), which were present during the earliest surveys but peaked in relative occurrence in late June and disappeared from the plankton shortly thereafter (Supplementary Figs. S11–S12). Both species were minor contributions to larval fish community structure. The next grouping consisted of several clusters (f, g, h, i) in which numerous species of fish larvae appeared over an extended period that varied among clusters, ranging from April to October (cluster h – American plaice (Hippoglossoides platessoides) and Atlantic cod (Gadus morhua)), April to August (cluster i – snailfish (Liparis spp.) and radiated shanny (Ulvaria subbifurcata)) (Supplementary Figs. S13–S16). These taxa grouped with less frequent taxa with long periods of occurrence from April to August (cluster g – redfish (Sebastes spp.) and winter flounder (Pseudopleuronectes americanus)) and June to October (cluster f – yellowtail founder (Myzopsetta ferruginea)) (Supplementary Figs. S17–S19). American plaice dominated at times whereas cod, snailfish, and radiated shanny were major contributors to the ichthyoplankton community (Fig. 8). Members of cluster g were moderate contributors to the larval community whereas yellowtail flounder was a minor taxon in the community (Fig. 8). Clusters f, g, h, i likely occurred across seasons from spring to fall and link with 72.8% (h, i), 66.6% (g) and 63.1% (f) similarity. The next group consisted of five taxa that occurred mostly from late June until September or October (cluster e – Atlantic herring (Clupea harengus), fourbeard rockling (Enchelyopus cimbrius), capelin (M. villosus), cunner (Tautogolabrus adspersus), and witch flounder (Glyptocephalus cynoglossus)), for which spawning likely takes place from June through August (Supplementary Figs. S20–S24). Clusters f, g, h, and i join with cluster e at 54.4% similarity. Herring and rockling were moderate components of the larval community, witch flounder was a major element, and capelin and cunner were dominant species (Fig. 8). The final group (cluster d – lumpfish (Cyclopterus lumpus) and Atlantic mackerel (Scomber scombrus)) occurred in very low overall relative numbers during restricted periods from late June to late August (Supplementary Figs. S25–S26). The period of occurrence for lumpfish may represent a dispersal window following emergence of the larvae from the nests, normally guarded by the males, whereas the occurrence of Atlantic mackerel aligns with the seasonal migration of adults along with limited spawning activity in coastal Newfoundland. Both lumpfish and mackerel were minor contributors to the ichthyoplankton community. Cluster d linked with other summer occurring taxa with 34.3% similarity. All cluster linkages reported above were statistically significant (p < 0.05 or less), based on 999 permutations. The seasonal succession of taxa also revealed a negative, significant correlation between the average day of maximum occurrence and egg diameter (r = -0.51, p <0.05, n = 19), whereby species with larger eggs spawned earlier in the year.
Fig. 7
Species composition and community structure
Average species composition strongly varied seasonally and differed greatly among bays (Fig. 8). In all bays, larval fish density appeared at low levels in the spring and peaked in August, although low densities were present during July in Placentia Bay. One of two species of larval fish generally dominated Placentia Bay, with the greatest complexity in June when the larval assemblage shifted from sand lance domination to a community shared primarily by capelin and cunner. One or two species dominated the ichthyoplankton community in Conception Bay in April/May (blenny and Arctic shanny, respectively) and August/September (capelin, cunner, and witch flounder), but greater complexity and changes in species occurred in June and July, during which 19 and 22 taxa occurred, respectively. High densities of American plaice, Atlantic herring, winter flounder, and radiated shanny occurred in Conception Bay in June, followed by increasing importance of capelin larvae in July. Larval fish species composition in Trinity Bay distinctly differed from that of Placentia and Conception Bays in that Arctic shanny, blenny, sculpin, and sand lance dominated in May, followed by the continued presence of Arctic shanny and growing importance of American plaice and Atlantic cod in June. However, in July, capelin became the dominant taxon and remained so well into October, when the relative importance of witch flounder, Atlantic herring, fourbeard rockling, and Atlantic cod increased, albeit at a time of lowest overall density of larvae. Larval fish species composition in Notre Dame and White Bays highlights a later production cycle of ichthyoplankton species relative to other bays, with very low densities throughout the summer but with a substantial increase in September, largely driven by capelin larval production. A complex mix of taxa in July suggests a combination of local spawning by coastal species and possibly larval import from offshore spawners. Sand lances, blenny, Atlantic cod and witch flounder dominated July samples, although redfish, Arctic and radiated shanny, and snailfish also contributed notably to the larval fish assemblage. Capelin only appeared in August, when they became the dominant taxon, although Atlantic cod, snailfish, and Arctic shanny remained important elements of the ichthyoplankton community. In September, capelin dominated the larval fish assemblages, with Atlantic cod as the next most abundant species. All the bays shared the common feature of relative dominance and high densities of capelin starting in July and persisting throughout the remainder of the summer and fall. Most taxa occurred in all the bays, with the exception of lumpfish in Placentia Bay and the northern bays, as well as winter flounder, gunnel, and Atlantic mackerel in the northern bays. Arctic cod only occurred in Conception and Placentia Bays. The limited seasonal coverage of Notre Dame and White Bays constrained our capacity to comment extensively on “missing” taxa but we note that 17 of the 22 taxa considered in this synthesis occurred in at least one sample.
Fig. 8
Principal coordinate analysis revealed striking similarities among bays in the progression of the seasonal cycle in the larval fish assemblage with positive loadings on PCoA 1 at the start of the larval production cycle and negative loadings in August, September, and October (Fig. 9). Notre Dame and White Bays assemblages in June resembled those in April and May in the more southerly bays, with average loadings in August very distant from those of other bays on PCoA 1 but similar to those of Placentia Bay on PCoA 2. The greatest differences among the bays appear on PCoA 2 and PCoA 3. Similar cycles on PCoA 1 and PCoA 2 characterize pre- and post-collapse Conception Bay and pre-collapse Trinity Bay from May to September with the greatest differentiation in July. However, major changes in the seasonal larval production cycle during the post-collapse period in Trinity Bay, with community loading on PCoA 1 and PCoA 2 in June similar to that of the pre-collapse system in May, in contrast to a similar pre- and post-collapse July assemblage. The Placentia Bay larval production cycle on the first two principal coordinates distinctly differs from that of Conception and Trinity Bays, except in April and May, which likely reflects the strong influence of the continued presence in all bays of sand lance in June and the high contributions of cunner and capelin in July and August. Placentia Bay also differed from the other bays as a result of the lower overall abundance of commercial groundfish species, likely a reflection of the post-collapse state of the ecosystem on the south coast of Newfoundland. PCoA 3 likely characterizes differences in the occurrence of relatively less abundant species among the bays that we cannot synthesize succinctly (see species-specific cycles). The species loadings on the first two principal coordinates demonstrate the general seasonal succession of species. Winter/spring spawners (e.g., Arctic shanny, sculpin) load positively on PCoA 1 and PCoA 2, spring/summer spawners (e.g., Atlantic cod, American plaice, radiated shanny) load positively on PCoA 1 and negatively on PCoA 2, and late summer/fall spawners (e.g., cunner, witch flounder, capelin) load negatively on PCoA 1 with low loadings on PCoA 2. The strongest influence on PCoA 3 reflects differences in the relative abundance of Arctic shanny, Atlantic cod, American plaice, and radiated shanny among the bays, with relatively minor differences among other taxa.
Fig. 9
PERMANOVA analysis of fixed effects of bay and month, which partitions the variance among factors based on Euclidean distances to assess multivariate dispersion among groups, revealed highly significant (p < 1.001) effects of bay, month and month·bay interactions, explaining 30.7% of the total variance in larval fish assemblages, based on 999 permutations and Type III sums of squares. Month accounted for 19.7% of the variance, whereas month·bay interactions accounted for 7.2%, and differences among bays accounted for 3.8%. These results confirm the patterns noted in the principal coordinates analyses that revealed strong similarity in seasonal cycles in larval production, but which differed slightly among bays in terms of timing of occurrence of different taxa. All pairwise comparisons among bay, including pre- and post-collapse comparisons within Conception and Trinity Bays, were highly significantly different (p < 0.001), as were comparisons among months. Two-way analysis of similarities (ANOSIM) did not fully confirm this result in that it detected no significant differences among pre- and post collapse larval fish communities in Conception Bay (p 0.3), and no significant difference between larval fish communities in Trinity and Conception Bays during the post-collapse period (p 0.6). All other pairwise comparisons indicated highly significant differences. The ANOSIM global R value for the comparisons among bays was 0.213 ((p < 0.001) and the global R value for the comparisons among months was 0.315 (p < 0.001), based on 999 permutations. The differences in the outcomes may reflect that PERMANOVA tests for differences between groups whereas ANOSIM tests whether distances between groups are greater than within groups. The high variability in larval community structure at any given time may have resulted in the lack of differentiation in Conception Bay between ecosystem states.
SIMPER analyses based on Euclidian distances of log-transformed abundance data revealed that capelin always drove differences among bays (average 54.1% contribution to the comparisons, range 34.7–78.9) (Table 2). The next most important taxa contributed an average of 19.1% of the dissimilarity (range 7.52–31.76) but species varied among comparisons. American plaice was the second most important contributor to comparisons of Trinity Bay pre-collapse with other areas. Cunner was important in most of the other comparisons among bays, with the exception of the comparison between Trinity Bay and the northern bays, where Atlantic herring was more important. Witch flounder and Atlantic cod contributed to differences among most systems. Similar average capelin densities characterized pre- and post collapse periods in Conception and Trinity Bays but their occurrence was delayed temporally during the post-collapse period. Witch flounder was less abundant following the collapse whereas radiated shanny and cunner were more abundant. Atlantic cod, American plaice, and witch flounder were important contributors to dissimilarities between pre- and post-collapse larval fish communities of Trinity Bay, with all abundances substantially lower post-collapse. Capelin abundances were nonetheless similar in Trinity Bay, although with a delayed seasonal cycle, as seen in Conception Bay.
Table 2
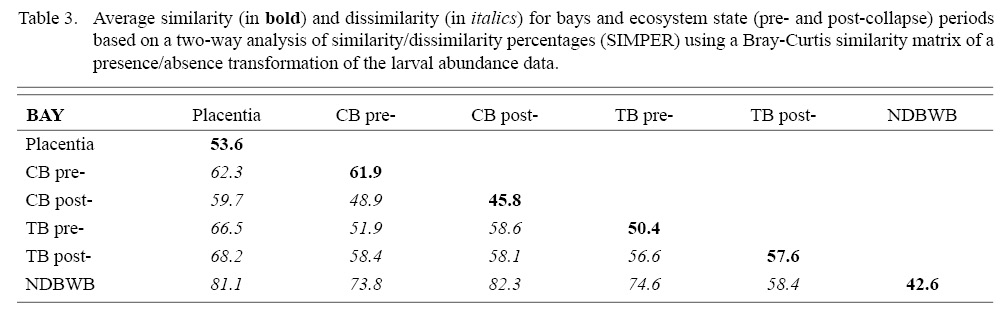
Table 3
SIMPER analyses based on Bray-Curtis similarity of presence/absence substantially reduced the influence of capelin on comparisons, and no single taxon had a highly dominant effect on dissimilarity in comparisons between bays. Average dissimilarity ranged from 48.9 (Conception Bay pre- and post-collapse) to 82.3 (Conception Bay post-collapse with Notre Dame and White Bays) (Table 3). Four to six species were required to account for at least 50% of the dissimilarity between bays. Occurrences of sand lance, cunner, American plaice, radiated shanny, and snailfish were the more important taxa in differentiating the Placentia Bay community from other bays. Higher densities and occurrence of American plaice, radiated shanny, along with yellowtail, witch, and winter flounders tended to characterize pre-collapse larval fish assemblages in Conception Bay relative to other bays. Conception Bay post-collapse generally differed from other bays, with greater average densities of American plaice, radiated shanny, snailfish, and lower densities of cod. Trinity Bay during the pre-collapse period generally differed from other bays with higher densities and occurrence of Atlantic cod, American plaice, capelin and lower densities of winter and yellowtail flounders. Several species of larval fish were depleted in post-collapse Trinity Bay with the exception of capelin, which appeared in greater abundance and frequency than in other bays. Atlantic cod and capelin densities and occurrence in Notre Dame and White Bays were comparable to other systems but the relative absence or infrequency of several species contributed substantially to differentiating these systems from other bays. Comparison of Conception Bay between the periods of differing ecosystem state indicates a decline in the occurrence of winter and witch flounders, and Atlantic cod, and increases in snail fish, capelin, radiated shanny, redfish, and blennies (Fig. 10). Comparison of Trinity between pre- and post-collapse periods revealed substantial declines in American plaice, Atlantic cod, witch and winter flounders, radiated shanny, snailfish, and redfish, along with modest increases in Arctic shanny and Atlantic herring, but similar levels of occurrence of capelin and cunner (Fig. 10). Changes in average abundance in Trinity Bay were generally more pronounced than those in Conception Bay.
Fig. 10
Species specific cycles
The presence of larvae close to the size at hatch later in the year than previously noted by Scott and Scott (1988) were noted for gunnel, sand lance, blenny, snailfish, winter flounder, radiated shanny, American plaice, yellowtail flounder, Atlantic cod, and capelin, possibly indicative of protracted spawning activity relative to previous knowledge (Table 4, Supplementary Material). The notable presence in April and May of large larvae of blenny, gunnel, sculpin, snailfish and Arctic shanny may indicate spawning in winter or early spring (Table 4, Supplementary Material). The presence in April and May of large larvae of Atlantic herring and capelin is likely indicative that fish spawned in the previous year overwintered in coastal waters.
Table 4
Overall occurrence patterns across all bays did not change consistently during the post-collapse period among fish taxa (Table 4, Supplementary Material), with increases in six taxa, declines in five, and no change in the remaining eleven taxa, although some patterns of change were location dependent (Supplementary Material and Figs. S5–S26). Post-collapse densities declined in 13 taxa, with important declines in commercially exploited species (Atlantic cod, American plaice, yellowtail flounder, winter flounder and lumpfish), but notable declines occurred in other demersal species not subjected to directed fisheries (radiated shanny, snailfish, blenny and fourbeard rockling) as well as one commercially targeted species (witch flounder) (Table 4). All forage species (capelin, Atlantic herring, and Atlantic mackerel) declined after the collapse of the Newfoundland commercial groundfish fisheries, but the extent of the declines varied spatially (Table 4, Supplementary Material). There were no changes in the abundance of eight non-commercial taxa (Table 4, Supplementary Material), although we note that adults of many of these taxa occur principally in habitats close to the coast and in relatively shallow waters (< 50 m), where fishery impacts caused by trawls may have been limited. A χ2 test revealed no significant difference in the distribution of egg types between taxa that declined in abundance versus those that did not change in abundance (p > 0.5), and a two-tailed t-test found no significant difference in average egg diameter between the two groups (p > 0.3).
Discussion
Our analyses demonstrate the value of long-term ichthyoplankton monitoring in quantifying changes in community structure from coastal Newfoundland, particularly those associated with the shifts in adult fish communities that resulted from either overexploitation, or changes in environmental conditions (Koslow and Wright, 2016; Gallo et al., 2019). Clear seasonal succession in species occurrence and composition were apparent in nearly all the bays included in our study, but this pattern reflected the relative mixture of taxa representing local differences in demersal and pelagic species community structure from adjacent continental shelves, and the latitudinal differences in the seasonal progression in environmental conditions. Furthermore, the data revealed longer spawning activity in many taxa than had previously been inferred from observations of gonadal development and condition in adult fish. Overall, these data provide a foundation from which to investigate possible changes in spawning activity and timing within each bay, identify expansion of species distributions in response to climate change, and monitor changes in the occurrence and abundance of taxa that standard scientific research trawl surveys may not effectively sample.
Precise timing of the collections during the year, spatiotemporal frequency of surveys, and differences in gear types confounded the various data sets in this synthesis, contributing to uncertainty in inferences derived from our analyses and assessments. However, comparable seasonal coverage prior to and following the collapse of several stocks should provide accurate representation of seasonal patterns in species occurrence and abundance. Overall, differences in community structure in ichthyoplankton composition were consistent with patterns reported in adult fish communities (Gomes et al., 1995; Pedersen et al., 2017), particularly the specific nature and magnitude of changes in ecosystem state. As for differences in gear types, Pepin and Shears (1997) demonstrated comparable catchabilities between the Tucker trawls used predominantly in Conception and Placentia Bays during the post-collapse period, and that of bongo nets, particularly for smaller and more abundant fish larvae. This inter-comparability would not have resulted in substantial errors in describing community structure because larger larvae would contribute less to overall catches for both gear types. Furthermore, Pepin and Shears (1997) found that Tucker trawl catches achieved greater precision in estimating the average abundance of fish eggs and larvae, although the abundance of very small larvae (1–3 mm) were sometimes less accurate for the Tucker trawl relative to bongo nets. In terms of occurrence, however, the patterns of change pre- and post-collapse indicated limited and variable changes for different taxa between periods. Furthermore, consistent declines in overall larval abundance of both commercial and non-commercial fish taxa were coherent with changes in adult fish community structure, suggesting that any potential bias in larval fish catchability resulting from differences in gear types did not affect the overall outcome of our synthesis. Consequently, the general patterns of occurrence and abundance described in our analyses are likely robust to potential uncertainty resulting from the differences in data sources.
Marked seasonality in environmental conditions and production of lower trophic levels characterize temperate, subarctic, and Arctic environments. Fish reproduction has adapted to release young to match availability of appropriate prey and thus meet larval metabolic requirements, known as the match-mismatch hypothesis (Cushing, 1969). Our analyses reveal that larval fish occur over a broad time period, starting in March–April, likely under the ice in some parts of coastal Newfoundland, until September–October. Relatively low densities at the start of the larval production cycle in April-May (~ 1 larva·m-2, range 0.01–10) quickly increase to ~100 larvae·m-2 (range 0.01–1 000) by late July, when larval capelin dominate the ichthyoplankton community. Larger egg diameters tend to characterize species that spawn early in the year, a pattern consistent with patterns of variation in egg diameter within and among species from the Gulf of St. Lawrence and the Scotian Shelf (Ware, 1977; Markle and Frost, 1985; Miller et al., 1995). This larger egg size has important implications for embryonic development rates (Pauly and Pullin, 1988; Pepin, 1991) and larval size (Bradbury et al., 2001), metabolic requirements (Moyano et al., 2018; Peck et al., 2021), ecological match between prey size and larval feeding capacity (Pepin and Penney, 1997; Pepin, 2023), and change in length required to reach metamorphosis (Chambers and Leggett, 1987; Benoit et al., 2000), which all relate to the seasonal environmental temperature cycle. Although absolute density and timing of peak abundance differed among bays, we observed relatively similar overall seasonal patterns in abundance and species composition over the limited latitudinal range covered by our synthesis. Lowest richness in the spring increased progressively to peak in July-August, declining thereafter to levels comparable to the spring. Higher species diversity in Conception and Trinity Bays relative to Placentia and the northern bays likely reflects the complexity of adult fish communities adjacent to Conception and Trinity Bays, and which transition into one another at the northern edge of the Grand Banks relative to areas further north or south of these systems (Mahon et al., 1998; Pepin et al., 2010; Pedersen et al., 2017). Median values of Shannon’s index of entropy, generally below 1 in most samples, coincides with uneven species composition across bays, and index values were highest in June and July when reproductive activity increases in many fish species. This pattern suggests more varied and richer larval communities during early summer months, when the copepod community, the principal prey of larval fish in the region (Pepin, 2023), increases most rapidly in diversity and composition (Maillet et al., 2022). Increased prey diversity may limit overlap in diet composition among species of larval fish, and thereby competition among larval fish species, although evidence from Conception Bay downplays the importance of food limitation in feeding and survival given high densities of small mesozooplankton in the coastal areas (Pepin and Penney, 2000; Tian et al., 2003). However, reproductive cycles in adult fish represent adaptations to conditions encountered at broader spatial scales representative of the stock’s or the species distribution rather than reflecting general features of local habitats that are not primary spawning locations (Lowerre-Barbieri et al., 2017). Instead, covariation in zooplankton diversity and ichthyoplankton species evenness in coastal Newfoundland likely reflects the transition from spring to early summer production of both communities that may result in favourable conditions for overall larval survival. Some differences in larval production cycles among bays likely also reflect latitudinal differences in the seasonal progression in oceanographic conditions and the reproductive cycles of local and adjacent adult fish populations.
Evaluating patterns of variation in beta diversity proved difficult because our analyses demonstrated strong dependency in metrics of local dissimilarity to the number of samples from the surveys included in this synthesis. Many of our process studies provided limited spatial coverage of each bay that resulted in large variability in turnover and nestedness of species composition among samples, which describe patterns of variation in replacement and inclusion of different taxa, particularly for surveys with fewer than 25 observations. The high variability in turnover and nestedness reflects the balance between larval sources within each bay (e.g., spawning beds, nest, habitat driven aggregations) and physical dispersal and mixing from currents, eddies, and areas of convergence and divergence in coastal Newfoundland (Pepin et al., 1995; Helbig and Pepin, 2002; Bradbury et al., 2003; Bradbury et al., 2008; Snelgrove et al., 2008; Pepin and Helbig, 2012). The asymptotic patterns in dissimilarity indicate limited (~3%) overall contribution of nestedness to beta diversity among samples, in which sites with smaller numbers of species represent subsets of the biotas at richer sites. This limited nestedness indicates horizontal mixing can substantially alter species composition among samples through species turnover, which involves the replacement of some species by others from site to site (Helbig and Pepin, 2002; Snelgrove et al., 2008). Dispersal and horizontal mixing play important roles in reproductive success of fish (Lowerre-Barbieri et al., 2017) and in connectivity among habitats and environments (Cowen et al., 2006). Previous studies in coastal Newfoundland emphasized the importance of transport and retention of larvae as drivers of ichthyoplankton distributions in relation to spawning locations to settlement or aggregation sites (Laprise and Pepin, 1995; Helbig and Pepin, 2002; Bradbury et al., 2003; Bradbury et al., 2008; Stanley et al., 2013; Murphy et al., 2018). The dynamic nature of currents in coastal bays of Newfoundland, and their vulnerability to short-term changes in atmospheric forcing (e.g., deYoung et al., 1993a; deYoung et al., 1993b), results in high variability in ichthyoplankton distributions in these systems, an issue highlighted by the large sample size required to accurately quantify variations in species composition of assemblages (i.e., beta diversity). Consequently, accurate monitoring of ichthyoplankton community structure and distribution in such ecosystems requires a significant investment in comprehensive sampling programs. Comprehensive sampling can avoid obscuring the response of fish populations or communities to changes in ecosystem state as a result of uncertainty in abundance estimates caused by vagaries in ocean circulation, and compounded by limited sample size (Pepin and Helbig, 2012). The limited differentiation in alpha and beta diversity of ichthyoplankton communities among coastal bays of Newfoundland indicates that similar underlying processes drive these embayments, both in terms of sources of larval fish and the physical dynamics of nearshore areas. These confounding processes often limit use of simple metrics of biodiversity to identify changes in coastal fish communities, and to infer changes in ecosystem functionality (Mori et al., 2018). Quantifying the drivers of biodiversity change in these complex systems will require in-depth evaluation of changes in the balance of occurrence and abundance of individual taxa to determine their role in overall community structure, and the influence of interactions among fish species.
Our synthesis revealed important similarities and differences among bays in the seasonal progression of the larval fish community structure, which indicates that no one location can serve as indicator of ecosystem state. Differences in adult species distributions along with vulnerabilities to environmental change and fishing activity have potentially important consequences to the survival potential of young fish from different parts of the coast. Our surveys revealed that major ecosystem change resulted in declines in larval abundance of commercially exploited species, and also declines in many non-commercial groundfish and pelagic species. We suggest limited likelihood that these changes resulted from by-catch in regional commercial fisheries, although we cannot discount the potential impact to small fish species resulting from increased shrimp trawling on the Newfoundland Shelf from the early 1990s onward (EJF, 2003). The magnitude and direction of change in abundance varied considerably among fish taxa following major regional changes in the ecosystem. For example, changes in abundance of capelin varied between pre and post collapse periods, whereas other taxa either increased in abundance (sand lance, redfish) or maintained similar densities (gunnel, sculpin, Arctic shanny, witch flounder, herring in Trinity Bay, and cunner). This variability suggests that, because of differences in life histories, not all taxa responded to ecosystem change in the same way. In the case of capelin, low stock biomass characterized the post-collapse period, along with delayed spawning and production of weaker year-classes despite a relatively high spawning extent (and larval densities) in some core areas (Trinity and Conception Bays) (Lewis et al., 2019; Murphy et al., 2021; Tripp et al., 2024). However, the limited basic research into the life history characteristics of other fish species in the region (e.g., Stares et al., 2007; Morgan et al., 2014; Morgan et al., 2018) constrains any inferences on how life history characteristics affected the varied response to changes in ecosystem state. Clearly, the densities of 13 of 22 fish taxa declined between pre- and post-collapse periods, reflecting important changes in the productivity of fish populations from the Newfoundland shelf and northern Grand Banks. Note, however, that the majority of taxa that demonstrated no change in abundance between pre- and post-collapse periods were principally those with habitats closely associated to the coast in relatively shallow waters, potentially limiting fishery impacts. Scientific trawl surveys also report important shifts in communities (Pedersen et al., 2017; Koen-Alonso and Cuff, 2018; Aune et al., 2024), and many coastal species not readily sampled by these surveys also decreased in abundance. However, assessing whether these declines resulted from unmonitored fishery impacts or from changes in lower trophic level productivity remains challenging. Chan et al. (2017) inferred long-term changes in primary productivity on the Labrador shelf from barium-to-calcium ratios and carbon isotopes from long-lived coralline algae, which they linked to the Atlantic Multidecadal Oscillation (AMO). They inferred that the AMO has been increasing since the late 1990s, as part of a prolonged Atlantic warming phase. Despite the continued positive phase in the AMO, our synthesis, and that of the broader fish and invertebrate community (Pedersen et al., 2017; Koen-Alonso and Cuff, 2018; Aune et al., 2024), indicates that the overall production potential has not resulted in a complete recovery to a pre-collapse state by the fish community on the Newfoundland shelf and northern Grand Banks. This recovery failure may indicate that ecosystem capacity and functionality may have changed between pre- and post-collapse periods, which could affect the sustainability of fisheries into the future.
A limited number of species often dominate the ichthyoplankton community in an area of interest (Auth and Brodeur, 2006; Campfield and Houde, 2011; Guan et al., 2017; Siddon et al., 2018). Our synthesis documented similar patterns, whether considered from a seasonal perspective or through differences in the seasonal succession among the four major coastal Newfoundland bays. The general dominance of capelin larvae from July until October in each of these bays was unusual relative to ichthyoplankton from other ecosystems. Capelin represent a keystone species on the Newfoundland shelf and Grand Banks (Buren et al., 2014; Buren et al., 2019). Like many other forage species (e.g., anchovy, herring, sardines, sprat), capelin larvae have an elongated body form, anguilliform swimming mode, and a relatively small mouth; they are vulnerable to starvation resulting partly from limited foraging capability (Peck et al., 2021; Moyano et al., 2023). Although previous studies cast significant doubt on food limitation for larval fish in coastal Newfoundland, even considering the high densities of capelin larvae (Pepin and Penney, 2000), the persistence of larger capelin larvae throughout the fall and winter months may result in local depletion of zooplankton during periods of low secondary productivity, although we were unable to find estimates of their potential impact. Randall et al. (2022) studied feeding patterns in Atlantic herring in Trinity Bay and concluded that they showed an adaptive feeding strategy, and O'Driscoll et al. (2001) found similar adaptive capacity in their analysis of adult and juvenile capelin diets. However, neither study evaluated the potential consumption rates of juvenile fish on their prey. Thus, the consequences of high capelin density, combined with that of other zooplanktivorous species that were once abundant, on coastal ecosystems and how this may impact survival and recruitment is unclear.
We detected no pre-/post-collapse differences in the occurrence or densities of capelin larvae in Trinity and Conception Bays despite important changes in larval survival potential (Penton and Davoren, 2013b; Murphy et al., 2018), and spawning characteristics (Penton and Davoren, 2013a; Murphy et al., 2021; Tripp et al., 2023), as well as much lower overall abundance of the stock (Buren et al., 2019; DFO, 2024) relative to that found during the pre-collapse period. The seasonal occurrence of capelin spawning starts on the south coast of Newfoundland and progressively moves later in the year as adult fish move in a northerly direction as the summer progresses (Nakashima, 1992; Murphy, 2022). Persistence of relatively high levels of larval abundance in Conception and Trinity Bays suggest that these two areas may represent core spawning locations. Any changes related to the recovery of the stock, despite current low levels of recruitment (Murphy et al., 2018; Murphy et al., 2021), could influence reproductive activities in other areas along the coast, or resulted in lengthened spawning periods across the range of the stock (Murphy, 2022). Alternatively, the high frequency of occurrence and dominance of capelin larvae in ichthyoplankton surveys, combined with the reduction in the number of samples collected and spatial coverage between pre- and post-collapse periods, could suggest bias in our findings about current states of the stock based on our opportunistic synthesis of survey information. Properly contrasting larval capelin abundance and its relationship with the adult population will require comprehensive sampling to characterize the reproductive potential of the Newfoundland capelin stock.
Combining multiple ichthyoplankton surveys provided a finer-resolution perspective of spawning activity in coastal Newfoundland that extended the periods of reproduction of half of the taxa included in our analyses relative to previous syntheses of information (Scott and Scott, 1988; Fahay, 1992a; Fahay, 1992b). This extended spawning season may, however, have minimal consequences for recruitment variability and population dynamics given that individuals spawned later in the season may lack sufficient energy reserves to survive periods with reduced productivity of lower trophic levels (Post and Parkinson, 2001; Laurel et al., 2003; Geissinger et al., 2023). This limitation may be a particularly important aspect of early life dynamics for capelin in which delayed beach spawning since the stock’s collapse has generally yielded weak year classes (Murphy et al. 2021). The consequences of protracted spawning activity to population dynamics and productivity will depend entirely on overall energetic demands on adults and the relative magnitude of egg and larval production later in the season, which are currently unknown for most species in the study region. However, spawning activity may provide an opportunity for certain taxa to benefit from the impacts of climate change, which will likely increase temperatures later in the season and thereby lengthen the period of enhanced plankton productivity (Han et al., 2015).
Conclusions
Combining data from multiple ichthyoplankton surveys conducted in coastal Newfoundland has provided important new knowledge about the seasonal larval production cycle across a broad range of species. Clearly, the consequences of major changes in commercial fish stocks resulting from overfishing and abrupt changes in environmental conditions also affected several non-commercial species found in coastal ecosystems. We achieved this finding despite imperfect overlap in the timing and occurrence of ichthyoplankton surveys. Our findings highlight the importance of basic biological knowledge of life history cycles of fish which is enabled by better observations of lower trophic levels in the form of pre-recruits. Determining whether such knowledge can serve to inform scientists about the underlying processes affecting multispecies dynamics from the Newfoundland shelf and Grand Banks will require further analysis.
Acknowledgements
The data for this study result from efforts by technicians, students, biologists, and scientists involved in the surveys and the processing of the samples. Staff from the Huntsman Marine Science Centre (St. Andrews, New Brunswick, Canada), particularly Lou van Guelpen and Gerhard Pohle, provided taxonomic expertise in the sorting and identification of ichthyoplankton from some of the samples. The officers and crew of Canadian Coast Guard Ships (CCGS) Marinus, Shamook, Teleost, Templeman and Vladykov, along with those of MV Mares, FV Fisherman’s Dream, and FV Twisted Sisters, were instrumental in providing support during the surveys. We appreciate Lauren Gullage’s efforts in the development of Fig. 1, and the contributions of climatological seasonal cycles of oceanographic conditions at Station 27 from Frédéric Cyr and David Bélanger. We appreciate the constructive comments from two anonymous reviewers, along with those of the handling editor, which resulted in improvements to the final manuscript.
Author contributions
PP conceptualized the study, carried out the analyses, and prepared the initial draft of the manuscript. All authors contributed data (collection, curation, quality control, and verification) for the synthesis, and participated in the writing, critical review, editing and revision of the manuscript.
Data availability
The data from this synthesis are available as an independent dataset from the corresponding author’s ResearchGate profile https://www.researchgate.net/profile/Pierre-Pepin/research
Conflict of interest
The authors have no conflict of interest to declare.
Ethics statement
The current study was based on existing data, therefore no ethical approval was needed.
References
Amani, M., Macdonald, C., Salehi, A., Mahdavi, S., and Gullage, M. 2022. Marine habitat mapping using bathymetric LiDAR data: a case study from Bonne Bay, Newfoundland. Water, 14: 3809. https://doi.org/10.3390/w14233809
Anderson, M. J., Gorley, R. N., and Clarke, K. R. 2008. PERMANOVA + for PRIMER. Guide to software and Statistical Methods, PRIMER-E, Plymouth.
Aune, M., Ramasco, V., Wells, N., Warren, M., Cyr, F., Pedersen, E. J., Koen-Alonso, M., et al. 2024. Taxonomic and functional-trait metrics track recovery of demersal fish and shrimp communities following system collapse. Frontiers in Marine Science, 11. https://doi.org/10.3389/fmars.2024.1237573
Auth, T. D., and Brodeur, R. D. 2006. Distribution and community structure of ichthyoplankton off the coast of Oregon, USA, in 2000 and 2002. Marine Ecology Progress Series, 319: 199–213. https://doi.org/10.3354/meps319199
2013. An overview of ichthyoplankton research in the northern California Current region: Contributions to ecosystem assessments and management. California Cooperative Oceanic Fisheries Investigations Reports, 54: 107–126.
Baselga, A. 2010. Partitioning the turnover and nestedness components of beta diversity. Global Ecology and Biogeography, 19: 134–143. https://doi.org/10.1111/j.1466-8238.2009.00490.x
2012. The relationship between species replacement, dissimilarity derived from nestedness, and nestedness. Global Ecology and Biogeography, 21: 1223–1232. https://doi.org/10.1111/j.1466-8238.2011.00756.x
Baselga, A., Orme, D., Villeger, S., De Bortoli, J., Leprieur, F., Logez, M., Martinez-Santalla, S., et al. 2023. betapart: Partitioning beta diversity into turnover and nestedness components. R package version 1.6 https://CRAN.R-project.org/package=betapar.
Batchelder, H. P., and Kim, S. 2008. Lessons learned from the PICES/GLOBEC Climate Change and Carrying Capacity (CCCC) Program and Synthesis Symposium. Progress in Oceanography, 77: 83–91. https://doi.org/10.1016/j.pocean.2008.03.003
Benoit, H. P., Pepin, P., and Brown, J. A. 2000. Patterns of metamorphic age and length in marine fishes, from individuals to taxa. Canadian Journal of Fisheries and Aquatic Sciences, 57: 856–869. https://doi.org/10.1139/f00-019
Betancur-R, R., Wiley, E. O., Arratia, G., Acero, A., Bailly, N., Miya, M., Lecointre, G., and Ortí, G. 2017. Phylogenetic classification of bony fishes. BMC Evolutionary Biology, 17: 162. https://doi.org/10.1186/s12862-017-0958-3
Beyer, J. E. 1989. Recruitment statiblity and survival: simple size-specific theory with examples of the early life dynamics of marine fish. Dana Report, 7: 45–147.
Boeing, W. J., and Duffy-Anderson, J. T. 2008. Ichthyoplankton dynamics and biodiversity in the Gulf of Alaska: responses to environmental change. Ecological Indicators, 8: 292–302. https://doi.org/10.1016/j.ecolind.2007.03.002
Bradbury, I. R., Laurel, B. J., Robichaud, D., Rose, G. A., Snelgrove, P. V. R., Gregory, R. S., Cote, D., and Windle, M. J. S. 2008. Discrete spatial dynamics in a marine broadcast spawner: re-evaluating scales of connectivity and habitat associations in Atlantic cod (Gadus morhua L.) in coastal Newfoundland. Fisheries Research, 91: 299–309. https://doi.org/10.1016/j.fishres.2007.12.006
Bradbury, I. R., Snelgrove, P. V. R., and Pepin, P. 2003. Passive and active behavioural contributions to patchiness and spatial pattern during the early life history of marine fishes. Marine Ecology Progress Series, 257: 233–245. https://doi.org/10.3354/meps257233
Buren, A. D., Koen-Alonso, M., Pepin, P., Mowbray, F., Nakashima, B., Stenson, G., Ollerhead, N., and Montevecchi, W. A. 2014. Bottom-up regulation of capelin, a keystone forage species. Plos One, 9. https://doi.org/10.1371/journal.pone.0087589
Buren, A. D., Murphy, H. M., Adamack, A. T., Davoren, G. K., Koen-Alonso, M., Montevecchi, W. A., Mowbray, F. K., et al. 2019. The collapse and continued low productivity of a keystone forage fish species. Marine Ecology Progress Series, 616: 155–170. https://doi.org/10.3354/meps12924
Campfield, P. A., and Houde, E. D. 2011. Ichthyoplankton community structure and comparative trophodynamics in an estuarine transition zone. Fishery Bulletin, 109: 1–19.
Chambers, R. C., and Leggett, W. C. 1987. Size and age at metamorphosis in marine fishes - an analysis of laboratory-reared winter flounder (Pseudopleuronectes americanus) with a review of variation in other species. Canadian Journal of Fisheries and Aquatic Science, 44: 1936–1947. https://doi.org/10.1139/f87-238
Chan, P., Halfar, J., Adey, W., Hetzinger, S., Zack, T., Moore, G. W. K., Wortmann, U. G., et al. 2017. Multicentennial record of Labrador Sea primary productivity and sea-ice variability archived in coralline algal barium. Nature communications, 8: 15543. https://doi.org/10.1038/ncomms15543
Clarke, K. R., and Gorley, R. N. 2006. Primer Version 6: User Manual and Tutorial, PRIMER-E, Plymouth.
Cowen, R. K., Paris, C. B., and Srinivasan, A. 2006. Scaling of connectivity in marine populations. Science, 311: 522–527. https://doi.org/10.1126/science.1122039
Cushing, D. H. 1969. Regularity of spawning season of some fishes. Journal Du Conseil, 33: 81–92. https://doi.org/10.1093/icesjms/33.1.81
Cyr, F., and Galbraith, P. S. 2021. A climate index for the Newfoundland and Labrador shelf. Earth Syst. Sci. Data, 13: 1807–1828. https://doi.org/10.5194/essd-13-1807-2021
Dalley, K. L., Gregory, R. S., Morris, C. J., and Cote, D. 2017. Seabed habitat determines fish and macroinvertebrate community associations in a subarctic marine coastal nursery. Transactions of the American Fisheries Society, 146: 1115–1125. https://doi.org/10.1080/00028487.2017.1347105
Davidson, F. J. M., Greatbatch, R. J., and de Young, B. 2001. Asymmetry in the response of a stratified coastal embayment to wind forcing. Journal of Geophysical Research-Oceans, 106: 7001–7015. https://doi.org/10.1029/2000JC900052
Davis, C. C. 1982. A preliminary quantitative study of the zooplankton from Conception Bay, insular Newfoundland, Canada. Internationale Revue Der Gesamten Hydrobiologie, 67: 713–747.
1986. A comparison of the zooplankton in two Newfoundland Bays with differing influences from major currents. Internationale Revue Der Gesamten Hydrobiologie, 71: 11–47. https://doi.org/10.1002/iroh.19860710103
de Queiroz, A. K. O., Gasalla, M. A., Laux, M., Oliveira, R. R. M., Santa Rosa, F. D., Schroder-Nielsen, A., Postuma, F. A., et al. 2024. Dietary metabarcoding of keystone sardine species reveals the importance of their ichthyoplankton prey in food webs of the southern Brazilian Bight fisheries. Fisheries Oceanography. https://doi.org/10.1111/fog.12668
deYoung, B., Greatbatch, R. J., and Forward, K. B. 1993a. Diagnostic coastal circulation model with application to Conception Bay, Newfoundland. Journal of Physical Oceanography, 23: 2617–2635. https://doi.org/10.1175/1520-0485(1993)023%3C2617:ADCCMW%3E2.0.CO;2
deYoung, B., Otterson, T., and Greatbatch, R. J. 1993b. The local and nonlocal response of Conception Bay to wind forcing. Journal of Physical Oceanography, 23: 2636–2649. https://doi.org/10.1175/1520-0485(1993)023%3C2636:TLANRO%3E2.0.CO;2
deYoung, B., and Sanderson, B. 1995. The circulation and hydrography of Conception Bay, Newfoundland. Atmosphere-Ocean, 33: 135–162. https://doi.org/10.1080/07055900.1995.9649528
DFO 2019. Re-evaluation of the Placentia Bay-Grand Banks area to identify ecologically and biologically significant areas. Canadian Science Advisory Secretariat Science Advisory Report, 2019/040: 30p. https://waves-vagues.dfo-mpo.gc.ca/library-bibliotheque/40818949.pdf
DFO 2023. Oceanographic conditions in the Atlantic zone in 2022. Canadian Science Advisory Secretariat Science Advisory Report, 2023/019: 35p. https://waves-vagues.dfo-mpo.gc.ca/library-bibliotheque/41188792.pdf
DFO 2024. Assessment of divisions 2J+3KL capelin in 2022 and evaluation of proposed limit reference points. Canadian Science Advisory Secretariat Science Advisory Report, 2024/004: 16p. https://waves-vagues.dfo-mpo.gc.ca/library-bibliotheque/41230449.pdf
Dickey-Collas, M., Somarakis, S., Witthames, P. R., van Damme, C. J. G., Uriarte, A., Lo, N. C. H., and Bernal, M. 2012. Where do egg production methods for estimating fish biomass go from here? Fisheries Research, 117: 6–11. https://doi.org/10.1016/j.fishres.2012.01.002
EJF 2003. Squandering the Seas: How shrimp trawling is threatening ecological integrity and food security around the world. Environmental Justice Foundation, London, U.K.
Evans, G. T., and Pepin, P. 1989. Potential for prediction plankton populations (and fish recruitment) from environmental data. Canadian Journal of Fisheries and Aquatic Sciences, 46: 898–903. https://doi.org/10.1139/f89-115
Fahay, M. P. 1983. A guide to the early stages of marine fishes occurring in the western North Atlantic Ocean, Cape Hatteras to the southern Scotian Shelf. Journal of the Northwest Atlantic Fisheries Organization, 4: 415 p. https://doi.org/10.2960/J.v4.a1
Fahay, M. P. 1992a. Early Stages of Fishes in the Western North Atlantic Ocean (Davis Strait, Southern Greenland and Flemish Cap to Cape Hatteras). Volume 1. Acipenseriformes through Syngnathiformes., Northwest Atlantic Fisheries Organization Special Issue. 931 pp.
Fahay, M. P. 1992b. Early Stages of Fishes in the Western North Atlantic Ocean (Davis Strait, Southern Greenland and Flemish Cap to Cape Hatteras). Volume 2. Scorpaeniformes through Tetraodontiformes., Northwest Atlantic Fisheries Organization Special Issue. 762 pp.
Fissel, B. E., Lo, N. C. H., and Herrick, S. F., Jr. 2011. Daily egg production, spawning biomass and recruitment for the central subpopulation of northern anchovy 1981–2009. California Cooperative Oceanic Fisheries Investigations Reports, 52: 116–135.
Fogarty, M. J., Botsford, L. W., and Werner, F. E. 2013. Legacy of the US GLOBEC program: current and potential contributions to marine ecosystem-based management. Oceanography, 26: 116–127. https://doi.org/10.5670/oceanog.2013.79
Froese, R., and Pauly, D. 2024. FishBase. World Wide Web electronic publication. www.fishbase.org, version (02/2024).
Frost, K. J., and Lowry, L. F. 1983. Demersal fishes and invertebrates trawled in the northeastern Chukchi and western Beaufort seas, 1976–77. NOAA Technical Report NMFS SSRF-764: 22p.
Gallo, N. D., Drenkard, E., Thompson, A. R., Weber, E. D., Wilson-Vandenberg, D., McClatchie, S., Koslow, J. A., and Semmens, B. X. 2019. Bridging from monitoring to solutions-based thinking: lessons from CalCOFI for understanding and adapting to marine climate change impacts. Frontiers in Marine Science, 6. https://doi.org/10.3389/fmars.2019.00695
Geissinger, E. A., Bloom, C. R., Gregory, R. S., Laurel, B. J., and Snelgrove, P. V. R. 2023. Condition, size, and winter duration affect winter survival probability of juvenile Atlantic cod Gadus morhua in a coastal subarctic ecosystem. Marine Ecology Progress Series, 711: 47–60. https://doi.org/10.3354/meps14310
Goldstein, E. D., Duffy-Anderson, J. T., Matarese, A. C., and Stockhausen, W. T. 2019. Larval fish assemblages in the eastern and western Gulf of Alaska: patterns, drivers, and implications for connectivity. Deep-Sea Research Part Ii-Topical Studies in Oceanography, 165: 26–40. https://doi.org/10.1016/j.dsr2.2018.09.003
Gomes, M. C., Haedrich, R. L., and Villagarcia, M. G. 1995. Spatial and temporal changes in the groundfish assemblages on the northeast Newfoundland Labrador Shelf, northwest Atlantic, 1978–1991. Fisheries Oceanography, 4: 85–101. https://doi.org/10.1111/j.1365-2419.1995.tb00065.x
Grant, S. M., and Brown, J. A. 1998. Nearshore settlement and localized populations of Atlantic cod (Gadus morhua) in shallow coastal waters of Newfoundland. Canadian Journal of Fisheries and Aquatic Sciences, 55: 1317–1327. https://doi.org/10.1139/f97-310
Guan, L., Dower, J. F., McKinnell, S. M., Pepin, P., Pakhomov, E. A., and Hunt, B. P. V. 2017. Interannual variability in the abundance and composition of spring larval fish assemblages in the Strait of Georgia (British Columbia, Canada) from 2007 to 2010. Fisheries Oceanography, 26: 638-654. https://doi.org/10.1111/fog.12223
Han, G. Q., Colbourne, E., Pepin, P., and Xie, Y. D. 2015. Statistical Projections of Ocean Climate Indices off Newfoundland and Labrador. Atmosphere-Ocean, 53: 556–570. https://doi.org/10.1080/07055900.2015.1047732
Hare, J. A., and Cowen, R. K. 1997. Size, growth, development, and survival of the planktonic larvae of Pomatomus saltatrix (Pisces: Pomatomidae). Ecology, 78: 2415–2431. https://doi.org/10.2307/2265903
Harris, L. 1990. Independent review of the state of the northern cod stock. https://waves-vagues.dfo-mpo.gc.ca/library-bibliotheque/114276.pdf
Helbig, J. A., and Pepin, P. 2002. The effects of short space and time scale current variability on the predictability of passive ichthyoplankton distributions: an analysis based on HF radar observations. Fisheries Oceanography, 11: 175–188. https://doi.org/10.1046/j.1365-2419.2002.00195.x
Houde, E. D. 1987. Fish early life dynamics and recruitment variability. American Fisheries Society Symposium, 2: 17–29.
Houde, E. D. 2008. Emerging from Hjort's shadow. Journal of Northwest Atlantic Fisheries Organization, 41: 53–70. https://doi.org/10.2960/J.v41.m634
Ings, D. W., Gregory, R. S., and Schneider, D. C. 2008. Episodic downwelling predicts recruitment of Atlantic cod, Greenland cod and white hake to Newfoundland coastal waters. Journal of Marine Research, 66: 529–561. https://doi.org/10.1357/002224008787157476
Islam, M. S., Ueno, M., and Yamashita, Y. 2015. Recruitment in Japanese sea bass, Lateolabrax japonicas (Cuvier, 1828): effects of timing on spawning and larval quality and quantity. Journal of Applied Ichthyology, 31: 1064–1071. https://doi.org/10.1111/jai.12861
Koen-Alonso, M., and Cuff, A. 2018. Status and trends of the fish community in the Newfoundland Shelf (NAFO Div. 2J3K), Grand Bank (NAFO Div. 3LNO) and Southern Newfoundland Shelf (NAFO Div. 3Ps) Ecosystem Production Units. NAFO Scientific Council Research Document, 18/070: 11p. https://www.nafo.int/Portals/0/PDFs/sc/2018/scr18-070.pdf
Koslow, J. A., and Wright, M. 2016. Ichthyoplankton sampling design to monitor marine fish populations and communities. Marine Policy, 68: 55–64. https://doi.org/10.1016/j.marpol.2016.02.011
Laprise, R., and Pepin, P. 1995. Factors influencing the spatio-temporal occurrence of fish eggs and larvae in a northern, physically dynamic coastal environment. Marine Ecology Progress Series, 122: 73–92. https://doi.org/10.3354/meps122073
Laurel, B. J., Gregory, R. S., and Brown, J. A. 2003. Settlement and distribution of age-0 juvenile cod, Gadus morhua and G. ogac, following a large-scale habitat manipulation. Marine Ecology Progress Series, 262: 241–252. https://doi.org/10.3354/meps262241
Legendre, P., and Gallager, E. D. 2001. Ecologically meaningful transformations for ordination of species data. Oecologia, 129: 271–280. https://doi.org/10.1007/s004420100716
Leggett, W. C., Frank, K. T., and Carscadden, J. E. 1984. Meteorological and hydrographic regulation of year-class strength in capelin (Mallotus villosus). Canadian Journal of Fisheries and Aquatic Sciences, 41: 1193–1201. https://doi.org/10.1139/f84-141
Lehodey, P., Alheit, J., Barange, M., Baumgartner, T., Beaugrand, G., Drinkwater, K., Fromentin, J. M., et al. 2006. Climate variability, fish, and fisheries. Journal of Climate, 19: 5009–5030. https://doi.org/10.1175/JCLI3898.1
Lewis, K. P., Buren, A. D., Regular, P. M., Mowbray, F. K., and Murphy, H. M. 2019. Forecasting capelin Mallotus villosus biomass on the Newfoundland shelf. Marine Ecology Progress Series, 616: 171–183. https://doi.org/10.3354/meps12930
Lilly, G. R., Nakken, O., and Brattey, J. 2013. A review of the contributions of fisheries and climate variability to contrasting dynamics in two Arcto-boreal Atlantic cod (Gadus morhua) stocks: persistent high productivity in the Barents Sea and collapse on the Newfoundland and Labrador Shelf. Progress in Oceanography, 114: 106–125. https://doi.org/10.1016/j.pocean.2013.05.008
Loder, J. W., Petrie, B. D., and Gawarkiewicz, G. 1998. The coastal ocean off northeastern North America: a large-scale view. In The Global Coastal Ocean: Regional Studies and Synthesis, pp. 105–133. Ed. by K. H. Brink, and A. R. Robinson. John Wiley, Hoboken, N. J.
Lowerre-Barbieri, S., DeCelles, G., Pepin, P., Catalan, I. A., Muhling, B., Erisman, B., Cadrin, S. X., et al. 2017. Reproductive resilience: a paradigm shift in understanding spawner-recruit systems in exploited marine fish. Fish and Fisheries, 18: 285–312. https://doi.org/10.1111/faf.12180
Ma, Z., Han, G., and deYoung, B. 2012. Modelling temperature, currents and stratification in Placentia Bay. Atmosphere-Ocean, 50: 244–260. https://doi.org/10.1080/07055900.2012.677413
Mahon, R., Brown, S. K., Zwanenburg, K. C. T., Atkinson, D. B., Buja, K. R., Claflin, L., Howell, G. D., et al. 1998. Assemblages and biogeography of demersal fishes of the east coast of North America. Canadian Journal of Fisheries and Aquatic Sciences, 55: 1704–1738. https://doi.org/10.1139/f98-065
Maillet, G., Belanger, D., Doyle, G., Robar, A., Rastin, S., Ramsay, D., and Pepin, P. 2022. Optical, chemical and biological oceanographic conditions on the Newfoundland and Labrador Shelf during 2018. Canadian Science Advisory Secretariat Research Document, 2022/075: viii + 53p.
Markle, D. F., and Frost, L. A. 1985. Comparative morphology, seasonality, and a key to planktonic fish eggs from the Nova Scotian Shelf. Canadian Journal of Zoology, 63: 246–257. https://doi.org/10.1139/z85-038
Marshall, K. N., Duffy-Anderson, J. T., Ward, E. J., Anderson, S. C., Hunsicker, M. E., and Williams, B. C. 2019. Long-term trends in ichthyoplankton assemblage structure, biodiversity, and synchrony in the Gulf of Alaska and their relationships to climate. Progress in Oceanography, 170: 134–145. https://doi.org/10.1016/j.pocean.2018.11.002
May, C. J., Ludsin, S. A., Glover, D. C., and Marschall, E. A. 2020. The influence of larval growth rate on juvenile recruitment in Lake Erie walleye (Sander vitreus). Canadian Journal of Fisheries and Aquatic Sciences, 77: 548–555. https://doi.org/10.1139/cjfas-2019-0059
Miller, T. J., Herra, T., and Leggett, W. C. 1995. An individual-based analysis of the variability of eggs and their newly-hatched larvae of Atlantic cod (Gadus morhua) on the Scotian Shelf. Canadian Journal of Fisheries and Aquatic Sciences, 52: 1083–1093. https://doi.org/10.1139/f95-106
Möllmann, C., Muller-Karulis, B., Kornilovs, G., and St John, M. A. 2008. Effects of climate and overfishing on zooplankton dynamics and ecosystem structure: regime shifts, trophic cascade, and feedback loops in a simple ecosystem. Ices Journal of Marine Science, 65: 302–310. https://doi.org/10.1093/icesjms/fsm197
Morgan, M. J., Koen-Alonso, M., Rideout, R. M., Buren, A. D., and Parsons, D. M. 2018. Growth and condition in relation to the lack of recovery of northern cod. Ices Journal of Marine Science, 75: 631–641. https://doi.org/10.1093/icesjms/fsx166
Morgan, M. J., Shelton, P. A., and Rideout, R. M. 2014. Varying components of productivity and their impact on fishing mortality reference points for Grand Bank Atlantic cod and American plaice. Fisheries Research, 155: 64–73. https://doi.org/10.1016/j.fishres.2014.02.019
Mori, A. S., Isbell, F., and Seidl, R. 2018. β-Diversity, Community Assembly, and Ecosystem Functioning. Trends in Ecology & Evolution, 33: 549–564. https://doi.org/10.1016/j.tree.2018.04.012
Morson, J. M., Grothues, T. M., and Able, K. W. 2019. Change in larval fish assemblage in a USA east coast estuary estimated from twenty-six years of fixed weekly sampling. Plos One, 14: e0224157. https://doi.org/10.1371/journal.pone.0224157
Moyano, M., Illing, B., Akimova, A., Alter, K., Bartolino, V., Börner, G., Clemmesen, C., et al. 2023. Caught in the middle: bottom-up and top-down processes impacting recruitment in a small pelagic fish. Reviews in Fish Biology and Fisheries, 33: 55–84. https://doi.org/10.1007/s11160-022-09754-3
Moyano, M., Illing, B., Christiansen, L., and Peck, M. A. 2018. Linking rates of metabolism and growth in marine fish larvae. Marine Biology, 165. https://doi.org/10.1007/s00227-017-3252-4
Murphy, H. M. 2022. Capelin beach spawning diaries: an analysis of 30 years of citizen science data from the island of Newfoundland, Canada. Cybium, 46: 257–370.
Murphy, H. M., Adamack, A. T., and Cyr, F. 2021. Identifying possible drivers of the abrupt and persistent delay in capelin spawning timing following the 1991 stock collapse in Newfoundland, Canada. Ices Journal of Marine Science, 78: 2709–2723. https://doi.org/10.1093/icesjms/fsab144
Murphy, H. M., Pepin, P., and Robert, D. 2018. Re-visiting the drivers of capelin recruitment in Newfoundland since 1991. Fisheries Research, 200: 1–10. https://doi.org/10.1016/j.fishres.2017.12.005
Myers, R. A., Hutchings, J. A., and Barrowman, N. J. 1997. Why do fish stocks collapse? The example of cod in Atlantic Canada. Ecological Applications, 7: 91–106. https://doi.org/10.1890/1051-0761(1997)007[0091:WDFSCT]2.0.CO;2
NAFO. 2023. Report of the 16th Meeting of the NAFO Scientific Council Working Group on Ecosystem Science and Assessment (WG-ESA) 14-23 November 2023. SCS Doc. 23/25. 76 pp. https://www.nafo.int/Portals/0/PDFs/sc/2023/scs23-25.pdf
Nakashima, B. S. 1992. Patterns in coastal migration and stock structure of capelin (Mallotus villosus). Canadian Journal of Fisheries and Aquatic Sciences, 49: 2423–2429. https://doi.org/10.1139/f92-268
Nielsen, J. M., Rogers, L. A., Brodeur, R. D., Thompson, A. R., Auth, T. D., Deary, A. L., Duffy-Anderson, J. T., et al. 2021. Responses of ichthyoplankton assemblages to the recent marine heatwave and previous climate fluctuations in several Northeast Pacific marine ecosystems. Global Change Biology, 27: 506–520. https://doi.org/10.1111/gcb.15415
O’Driscoll, R. L., Parsons, M. J. D., and Rose, G. A. 2001. Feeding of capelin (Mallotus villosus) in Newfoundland waters. Sarsia, 86: 165–176. https://doi.org/10.1080/00364827.2001.10420472
Oozeki, Y. 2018. Biological monitoring: Fish eggs, fish larvae, and zooplankton. In Fish population dynamics, monitoring, and management: sustainable fisheries in the eternal Ocean, pp. 111–138. Ed. by I. Aoki, T. Yamakawa, and A. Takasuka. Springer Japan, Tokyo. https://doi.org/10.1007/978-4-431-56621-2_7
Pauly, D., and Pullin, R. S. V. 1988. Hatching time in spherical, pelagic marine fish eggs in response to temperature and egg size. Environmental Biology of Fishes, 22: 261–271. https://doi.org/10.1007/BF00004892
Peck, M. A., Alheit, J., Bertrand, A., Catalán, I. A., Garrido, S., Moyano, M., Rykaczewski, R. R., et al. 2021. Small pelagic fish in the new millennium: A bottom-up view of global research effort. Progress in Oceanography, 191. https://doi.org/10.1016/j.pocean.2020.102494
Pedersen, E. J., Thompson, P. L., Ball, R. A., Fortin, M. J., Gouhier, T. C., Link, H., Moritz, C., et al. 2017. Signatures of the collapse and incipient recovery of an overexploited marine ecosystem. Royal Society Open Science, 4. https://doi.org/10.1098/rsos.170215
Penton, P. M., and Davoren, G. K. 2013a. Capelin (Mallotus villosus) fecundity in post-1990s coastal Newfoundland. Marine Biology, 160: 1625–1632. https://doi.org/10.1007/s00227-013-2215-7
Penton, P. M., and Davoren, G. K. 2013b. A common garden experiment on capelin (Mallotus villosus) early life history stages to examine use of beach and deep-water spawning habitats. Journal of Experimental Marine Biology and Ecology, 439: 54–60. https://doi.org/10.1016/j.jembe.2012.10.009
Pepin, P. 1991. Effect of temperature and size on development, mortality, and survival rates of pelagic early life history stages of marine fish. Canadian Journal of Fisheries and Aquatic Sciences, 48: 503–518. https://doi.org/10.1139/f91-065
Pepin, P. 2016. Reconsidering the impossible - linking environmental drivers to growth, mortality, and recruitment of fish. Canadian Journal of Fisheries and Aquatic Sciences, 73: 205–215. https://doi.org/10.1139/cjfas-2015-0091
Pepin, P. 2023. Feeding by larval fish: how taxonomy, body length, mouth size, and behaviour contribute to differences among individuals and species from a coastal ecosystem. Ices Journal of Marine Science, 80: 91–106. https://doi.org/10.1093/icesjms/fsac215
Pepin, P., Colbourne, E., and Maillet, G. 2011. Seasonal patterns in zooplankton community structure on the Newfoundland and Labrador Shelf. Progress in Oceanography, 91: 273–285. https://doi.org/10.1016/j.pocean.2011.01.003
Pepin, P., Cuff, A., Koen-Alonso, M., and Ollerhead, N. 2010. Preliminary analysis for the delineation of marine ecoregions on the Newfoundland and Labrador Shelves. 24 pp.
Pepin, P., Dower, J. F., and Davidson, F. J. M. 2003. A spatially explicit study of prey-predator interactions in larval fish: assessing the influence of food and predator abundance on larval growth and survival. Fisheries Oceanography, 12: 19–33. https://doi.org/10.1046/j.1365-2419.2003.00221.x
Pepin, P., and Helbig, J. A. 2012. Sampling variability of ichthyoplankton surveys-Exploring the roles of scale and resolution on uncertainty. Fisheries Research, 117: 137–145. https://doi.org/10.1016/j.fishres.2011.07.004
Pepin, P., Helbig, J. A., Laprise, R., Colbourne, E., and Shears, T. H. 1995. Variations in the contribution of transport to changes in planktonic animal abundance - A study of the flux of fish larvae in Conception Bay, Newfoundland. Canadian Journal of Fisheries and Aquatic Sciences, 52: 1475–1486. https://doi.org/10.1139/f95-142
Pepin, P., and Penney, R. W. 1997. Patterns of prey size and taxonomic composition in larval fish: are there general size-dependent models? Journal of Fish Biology, 51: 84–100. https://doi.org/10.1111/j.1095-8649.1997.tb06094.x
Pepin, P., and Penney, R. W. 2000. Feeding by a larval fish community: Impact on zooplankton. Marine Ecology Progress Series, 204: 199–212. https://doi.org/10.3354/meps204199
Pepin, P., and Shears, T. H. 1997. Variability and capture efficiency of bongo and Tucker trawl samplers in the collection of ichthyoplankton and other macrozooplankton. Canadian Journal of Fisheries and Aquatic Sciences, 54: 765–773. https://doi.org/10.1139/f96-347
Peterson, G. S., Hoffman, J. C., Trebitz, A. S., Hatzenbuhler, C. I., Myers, J. T., Ross, J. E., Okum, S. L., and Pilgrim, E. M. 2022. Early detection monitoring for non-indigenous fishes: comparison of survey approaches during two species introductions in a Great Lakes port. Biological Invasions, 24: 463–478. https://doi.org/10.1007/s10530-021-02655-9
Petrie, B., Akenhead, S., Lazier, J., and Loder, J. W. 1988. The cold intermediate layer on the northeast Newfoundland and Labrador Shelves, 1978–1986. NAFO Scientific Council Studies, 12: 57–69. https://www.nafo.int/Portals/0/PDFs/Studies/s12/petrie.pdf
Petrie, B., Loder, J. W., Akenhead, S., and Lazier, J. 1991. Temperature and salinity variability on the eastern Newfoundland Shelf - The annual harmonic. Atmosphere-Ocean, 29: 14–36. https://doi.org/10.1080/07055900.1991.9649390
Petrie, B., Loder, J. W., Lazier, J., and Akenhead, S. 1992. Temperature and salinity variability on the eastern Newfoundland Shelf - The residual field. Atmosphere-Ocean, 30: 120–139. https://doi.org/10.1080/07055900.1992.9649433
Post, J. R., and Parkinson, E. A. 2001. Energy allocation strategy in young fish: allometry and survival. Ecology, 82: 1040–1051. https://doi.org/10.1890/0012-9658(2001)082[1040:EASIYF]2.0.CO;2
Proudfoot, B., Devillers, R., Brown, C. J., Edinger, E., and Copelan, A. 2020. Seafloor mapping to support conservation planning in an ecologically unique fjord in Newfoundland and Labrador, Canada. Journal of Coastal Conservation, 24: 36. https://doi.org/10.1007/s11852-020-00746-8
R Core Team 2023. R 4.3.1: A language and environment for statistical computing. R Foundation for Statistical Computing, Vienna, Austria. Available online at https://www.R-project.org/.
Randall, J. R., Murphy, H. M., Robert, D., and Geoffroy, M. 2022. Forage fish as a predator: summer and autumn diet of Atlantic herring in Trinity Bay, Newfoundland. Fisheries Research, 252. https://doi.org/10.1016/j.fishres.2022.106331
Renkawitz, M. D., Gregory, R. S., and Schneider, D. C. 2011. Habitat dependant growth of three species of bottom settling fish in a coastal fjord. Journal of Experimental Marine Biology and Ecology, 409: 79–88. https://doi.org/10.1016/j.jembe.2011.08.004
Robert, D., Castonguay, M., and Fortier, L. 2007. Early growth and recruitment in Atlantic mackerel Scomber scombrus: discriminating the effects of fast growth and selection for fast growth. Marine Ecology Progress Series, 337: 209–219. https://doi.org/10.3354/meps337209
Robert, D., Takasuka, A., Nakatsuka, S., Kubota, H., Oozeki, Y., Nishida, H., and Fortier, L. 2010. Predation dynamics of mackerel on larval and juvenile anchovy: is capture success linked to prey condition? Fisheries Science, 76: 183–188. https://doi.org/10.1007/s12562-009-0205-y
Scott, W. B., and Scott, G. B. 1988. Atlantic fishes of Canada. Canadian Bulletin of Fisheries and Aquatic Sciences. 219. 731 pp.
Siddon, E. C., Duffy-Anderson, J. T., Mier, K. L., Busby, M. S., and Eisner, L. B. 2018. Seasonal, interannual, and spatial patterns of community composition over the eastern Bering Sea shelf in cold years. Part II: ichthyoplankton and juvenile fish. Ices Journal of Marine Science, 75: 87–101. https://doi.org/10.1093/icesjms/fsx123
Siddon, E. C., Duffy-Anderson, J. T., and Mueter, F. J. 2011. Community-level response of fish larvae to environmental variability in the southeastern Bering Sea. Marine Ecology Progress Series, 426: 225–239. https://doi.org/10.3354/meps09009
Simmonds, E. J. 2009. Evaluation of the quality of the North Sea herring assessment. Ices Journal of Marine Science, 66: 1814–1822. https://doi.org/10.1093/icesjms/fsp104
Snelgrove, P. V. R., Bradbury, I. R., de Young, B., and Fraser, S. 2008. Temporal variation in fish egg and larval production by pelagic and bottom spawners in a large Newfoundland coastal embayment. Canadian Journal of Fisheries and Aquatic Sciences, 65: 159–175. https://doi.org/10.1139/f07-148
Somarakis, S., Schismenou, E., Siapatis, A., Giannoulaki, M., Kallianiotis, A., and Machias, A. 2012. High variability in the daily egg production method parameters of an eastern Mediterranean anchovy stock: influence of environmental factors, fish condition and population density. Fisheries Research, 117: 12–21. https://doi.org/10.1016/j.fishres.2010.11.027
Stanley, R. R. E., deYoung, B., Snelgrove, P. V. R., and Gregory, R. S. 2013. Factors regulating early life history dispersal of Atlantic cod (Gadus morhua) from coastal Newfoundland. Plos One, 8. https://doi.org/10.1371/journal.pone.0075889
Stares, J. C., Rideout, R. M., Morgan, M. J., and Brattey, J. 2007. Did population collapse influence individual fecundity of northwest atlantic cod? Ices Journal of Marine Science, 64: 1338-1347. https://doi.org/10.1093/icesjms/fsm127
Takasuka, A., Sakai, A., and Aoki, I. 2017. Dynamics of growth-based survival mechanisms in Japanese anchovy (Engraulis japonicus) larvae. Canadian Journal of Fisheries and Aquatic Sciences, 74: 812–823. https://doi.org/10.1139/cjfas-2016-0120
Tian, R. C., Deibel, D., Thompson, R. J., and Rivkin, R. B. 2003. Modeling of climate forcing on a cold-ocean ecosystem, Conception Bay, Newfoundland. Marine Ecology Progress Series, 262: 1–17. https://doi.org/10.3354/meps262001
Tripp, A., Murphy, H. M., and Davoren, G. K. 2024. Evidence for adaptive strategies in larval capelin on the northeastern coast of Newfoundland, Canada. Journal of Plankton Research, 46(2): https://doi.org/10.1093/plankt/fbad052
Tripp, A., Murphy, H. M., Penton, P. M., and Davoren, G. K. 2023. Trends in larval densities, spawning timing, and site quality of capelin (Mallotus villosus) across bays and habitats in Newfoundland, Canada. Ices Journal of Marine Science. https://doi.org/10.1093/icesjms/fsad141
Voss, R., Peck, M. A., Hinrichsen, H. H., Clemmesen, C., Baumann, H., Stepputtis, D., Bernreuther, M., et al. 2012. Recruitment processes in Baltic sprat - A re-evaluation of GLOBEC Germany hypotheses. Progress in Oceanography, 107: 61–79. https://doi.org/10.1016/j.pocean.2012.05.003
Ware, D. M. 1977. Spawning time and egg size of Atlantic mackerel, Scomber scombrus, in relation to plankton. Journal of the Fisheries Research Board of Canada, 34: 2308–2315. https://doi.org/10.1139/f77-309
Wilson, C. J., Murphy, H. M., Bourne, C., Pepin, P., and Robert, D. 2018. Feeding ecology of autumn-spawned Atlantic herring (Clupea harengus) larvae in Trinity Bay, Newfoundland: Is recruitment linked to main prey availability? Journal of Plankton Research, 40: 255–268. https://doi.org/10.1093/plankt/fby0033
Worm, B., and Myers, R. A. 2003. Meta-analysis of cod-shrimp interactions reveals top-down control in oceanic food webs. Ecology, 84: 162–173. https://doi.org/10.1890/0012-9658(2003)084[0162:MAOCSI]2.0.CO;2
Yao, T. 1986. The response of currents in Trinity Bay, Newfoundland, to local wind forcing. Atmosphere-Ocean, 24: 235–252. https://doi.org/10.1080/07055900.1986.9649249
Citation: Pepin, P., Mowbray, F.K., Murphy, H.M., Robert, D., and Snelgrove, P.V.R. 2024. Seasonal succession of the larval fish community from coastal areas of eastern Newfoundland, Canada. J. Northw. Atl. Fish. Sci., 55: 31–58. https://doi.org/10.2960/J.v55.m749