J. Northw. Atl. Fish. Sci., Vol. 54: 17-29
Benjamin R. LaFreniere1,2, Rebecca Peters2, Briony Donahue2, Richard McBride3, and John A. Mohan1
1University of New England, School of Marine and Environmental Programs,
11 Hills Beach Rd, Biddeford ME 04005
2Maine Department of Marine Resources, 194 McKown Pt. Rd. West Boothbay Harbor 04575
3National Oceanic and Atmospheric Administration, Northeast Fisheries Science Center,
166 Water St, Woods Hole, MA 02543
LaFreniere, B.R., Peters R. Donahue, B., McBride, R., and Mohan, J.A. 2023. What the Hakes? Correlating Environmental Factors with Hake Abundance in the Gulf of Maine. J. Northw. Atl. Fish. Sci., 53: 17–29. https://doi.org/10.2960/J.v54.m742
Abstract
According to the latest estimates, the Gulf of Maine is currently warming faster than 99% of the world’s oceans. As a result, this region has become an ideal location for research into the effects that warming has on the historical fisheries that make up this ocean basin. Both white hake (Urophycis tenuis) and red hake (Urophycis chuss) are common Gulf of Maine groundfish species, distributed both inshore and offshore. While these two species are closely related phycid hakes, white hake stocks are recognized in the Gulf of Maine as rebuilding, while red hake are above target biomass levels. As a species commonly found throughout the Gulf of Maine that prefers cooler waters (4–12°C), we hypothesize the effects of climate change might influence stock behavior, such as changes in species distribution. We used generalized additive models (GAMs) to describe the relationship between hake abundance and environmental conditions using bottom temperature, bottom salinity, depth, and catch data contributed by the Maine Department of Marine Resources during their Maine – New Hampshire Inshore Trawl Surveys of the last 22 years (2000 – 2021). Our results reveal species-specific preferences for bottom temperature (white hake ~9 to ~13℃, red hake < 12℃) and depth (white hake ~55 to ~100m, red hake > ~65m), with no significant correlation to bottom salinity. Spatially over time, white hake abundance displayed a gradual center of gravity northward, while red hake rapidly increased inshore. Overall, these results highlight species-specific density changes in inshore distribution, consistent with previous studies, with considerable implications on future management strategies in this region.
Keywords hakes, environmental factors, Gulf of Maine, abundance, climate change
PDF
Download Citation Data

Citation to clipboard
Reference management software (Endnote, Mendeley, RefWords, Zotero & most other reference management software)

LaTex, BibDesk & other specific software
Introduction
The Gulf of Maine (GoM) is known historically for its commercial fisheries, including demersal Atlantic cod (Gadus morhua) and American lobster (Homarus americanus), and pelagic migratory Atlantic bluefin tuna (Thunnus thynnus) (Jones and Borque, 1998; Lotze and Milewski, 2004). These fisheries have been, and continue to be, a staple of New England’s economy and culture (Lotze and Milewski, 2004). However, in recent decades the GoM has been subject to record trends in warming, faster than reported 99% of the global ocean (Pershing et al., 2015), with signs of continued warming (Saba et al., 2016). These unprecedented temperature increases are alarming to fishery managers, as Pershing et al. (2015) directly correlated the decline of the Atlantic cod fishery to such warming. Despite speculation into the drivers of declining fish stocks, it is clear that increased warming in the GoM region will have major effects on New England communities, and native fishery species (Perry et al., 2005; Cheung et al., 2013; Mills et al., 2013, Pershing et al., 2021).
With this increasing awareness of the correlation between climate pressures and population dynamics, research into how climate change will affect the fish assemblages in the GoM have increased (Overholtz and Link, 2009; Gaichas et al.; 2014; Hare et al., 2016). While such investigations have proven useful to track this ecosystem, finer scale, species-specific investigations are still necessary. Species-specific investigations have been conducted on a range of species including American lobster, (Goode et al., 2019), Atlantic herring (Clupea harengus) (Moyano et al., 2020), Atlantic mackerel (Scomber scombrus) (Overholtz et al., 2011), Atlantic cod (Pershing et al., 2015; Fogarty et al., 2008), and northern shrimp (Pandalus borealis) (Richards et al., 2012). However, there is still a wide range of species yet to be investigated, including GoM hakes.
White hake are a historically important groundfish species in the GoM (Ames, 2012). Found primarily in muddy substrates along the continental shelf and slope (Musick 1974; Ames, 2012), this once prominent commercial species dominated the GoM in the 1960s (Fritz, 1965). Although primarily caught as bycatch, historical reports state white hake landings were at a time higher than both Atlantic cod and haddock (Melanogrammus aeglefinus) in the fall months (Fritz, 1965). This dominance however was short lived, with white hake showing a decrease in landings by 62% between 1992 and 1996 (Sosebee et al., 1998). In the last decade, white hake have been variously assessed as overfished and not subject to overfishing (Northeast Fisheries Science Center 2019, 2021), and not overfished and not subject to overfishing (Northeast Fisheries Science Center 2013, 2017, 2022). While white hake are common in New England commercial fisheries, they are not the only hake species found in the GoM.
Red hake (Urophycis chuss) are a sibling species to white hake. Unlike white hake, the red hake fishery is historically very small in the GoM (O'Brien et al.,1993), occasionally targeted as a bait species but primarily landed as bycatch (New England Fishery Management Council, 2022). Currently, the GoM red hake stock status is unknown (Northeast Fisheries Science Center, 2020a) with overexploitation unlikely (Northeast Fisheries Science Center, 2020b) and catch limits well above recent landings since a productive year class in 2014 (National Marine Fisheries Service and New England Fishery Management Council, 2021).
A comparison of these two species by Markle et al. (1982) theorized that both ontogenetic and interspecific environmental partitioning between species was found for a range of fundamental life history characteristics. For reproduction, both species show variation with white hake employing the “get big quick” strategy, while red hake employ the “get mature quick” strategy (Markle et al., 1982). These distinct strategies distinguish internal physiology of these species, focusing energy to contrasting life stages and at different rates to fulfill their respective reproductive strategies (Markle et al., 1982)
While both species begin life as plankton, red hake migrate from the pelagic environment as post-larvae much earlier than white hake (~30 mm compared to 80 mm respectively) (Musick 1974; Sosebee et al., 1998), settling at the bottom. At the bottom, red hake display a symbiotic relationship with sea scallops (Placopecten magellanicus, residing in the scallop’s mantle until they outgrow their host (~110–140 mm) (Musick, 1974; Markle et al., 1982). No accounts of a sea scallop and white hake symbiotic relationship have been recorded, making this relationship unique to red hake (Musick, 1974; Markle et al., 1982; Sosebee et al., 1998). Early juvenile habitat selection is distinct for both species, with white hake preferring nearshore eelgrass beds and estuaries (Sosebee et al., 1998), while red hake prefer sea scallop beds (Markle et al.,1982; Steiner et al., 1982; Haedrich, 2003). While they show early habitat separation, both later stage juveniles and adults have been shown to coexist in both inshore and offshore soft bottom habitats, with both species showing preference to moderate temperatures (4–12°C) (Musick, 1974; Markle et al., 1982). While white hake are widely dispersed from Florida to Newfoundland, red hake are more geographically concentrated in the mid-Atlantic bight and GoM (Musick, 1974).
The current lack of extensive ecological knowledge in later life stages, paired with the impending pressures of climate change in the GoM, results in uncertain future viability of a GoM hake fishery. Hare et al. (2012) included both species in their risk assessment analysis, finding both species to be experiencing high climate exposure (temperature increase), with the biological sensitivity of red hake being low, while white hake was moderate. Hare et al. (2012) included a combination of factors ranging from stock status, prey specificity, and mortality. This correlates with the findings of Adams et al. (2018), which suggests that fishing pressure, like climate change, can also drive density changes in species distributions. Recent stock volatility of white hake in the GoM (rapid increase and decrease in population size), coupled with continued warming introduces potential drivers of increased risk, ultimately causing distribution fluctuations. Both studies highlight the need for continued investigations over a long-term data series, in aims to highlight the changing sensitivity of vulnerable species.
For this study, we aimed to correlate white and red hake abundance to environmental variables (bottom temperature, bottom salinity, and depth) over the past 22 years. hile we do expect both hake species to have different environmental preferences, we hypothesize observable overlap in preference due to their close biological relationship. Based on documented habitat density changes in other species, as well as prior understanding of hake habitat preferences, we expect to observe northern, offshore movement through time for both species (Kleisner et al., 2017; Adams et al., 2018). Ultimately, we expect hakes to be affected in both abundance and distribution due to climate change, with white hake being affected adversely due to their recent volatile stock trends, similar to the findings of Hare et al. (2012), supported by the claims of Adams et al. 2018).
Methods
Data Collection
White and red hake abundance and environmental data from the Maine Department of Marine Resources (ME DMR) Maine New Hampshire Inshore Trawl Survey (MENH survey) were used in these analyses. This survey has been conducted since the fall of 2000, sampling out to the 12 nm line, from Seabrook, NH to the Canada-United States border. The survey consistently has sampled depth strata from 6.9–100 m, including depths >100 m (maximum = 223.1 m) starting in 2004 (Fig. 1). This fisheries independent, multispecies trawl survey specifically targets inshore waters of both Maine and New Hampshire to enhance knowledge of exploited and other fish populations. Furthermore, this survey is unique in that it samples areas where federal trawl surveys run by National Oceanic and Atmospheric Administration (NOAA) fisheries (Northeast Fisheries Science Center (NEFSC) offshore trawl survey) are unable to reach, providing data for inshore species distributions.
Fig. 1
The gear for this survey consists of a modified shrimp net, which has 5.1 cm mesh wings and a 2.5 cm mesh liner in the cod end. The foot rope is 17.4 m long, and the head rope is 21.3 m long, with 15.2 cm rubber cookies. This gear was chosen due to its light weight, aiming to minimize habitat disruption during sampling, as well as its ability to sample multiple species of different sizes. Fishers in this region collaborated with ME DMR on the trawl survey design and gear type. The target trawl is aimed to be 20 minutes, with an average of 18.8 ± 2.2 minutes covering 0.78 ± 0.12 nautical miles. In addition to physical characteristics of the catch being recorded (species, length frequencies, weight), environmental variables (bottom temperature, bottom salinity, and depth) were recorded using a SeaBird CTD (19plus) after the trawl net is recovered on deck. This analysis only includes trawls where all three environmental conditions (temperature, salinity, and depth) as well as catch were recorded, excluding those where data was missing (83 trawls). Length frequencies were conducted for both white and red hake, for all tows, up to ~125 individuals. In cases where total catch for a species was > ~125 individuals, a random subsample was taken later extrapolated to calculate the total catch. While these trawl surveys have been conducted both in the spring and fall, ~87% of white hake and ~82% of red hake sampled (by both weight and number caught) came from the fall survey and therefore only fall survey data was used in our analysis. This was to be expected based on the known seasonal distributions of hake in this region (Fahay and Able, 1989; Ames 2012) .
Generalized Additive Models
We compared environmental conditions collected during sampling to the overall biomass of both white and red hake sampled. Abundance is calculated by the following formula, where X represents either white or red hake:

The length of each tow (m) was recorded for each survey set, and the average wing spread (m2) of the net was previously calculated by ME DMR.
From the abundance calculation for each individual tow over the 22 years of sampling, generalized additive models (GAMs) using RStudio (version 4.1.2 using the mgcv package) were developed to relate hake abundance to each continuous environmental variable (bottom temperature, bottom salinity, depth). GAMs are extensions of generalized linear models (GLMs), where data does not fit a linear trend, but is rather more complex and nonlinear (Hastie and Tibshirani, 1987). The GAMs were fitted with the following equation:
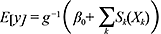
In the GAM equation, E[y] is the expected value of the response variable (in this case species abundance), g is the link function, β0 is the y-intercept, X is an explanatory variable (in this case a set of environmental conditions), k is the number of knots, and Sk is the smoothing function for the explanatory variables.
Due to identical number of sampling attempts and conditions for both species (multispecies sampling), we chose to run identical model conditions for both species, with only the dependent variables (species abundance) changing. We deemed that the high overlap in model conditions will allow us to compare these species over the last 22 years with increased confidence.
Before models were constructed, multicollinearity between environmental conditions were checked using the corrplot package in RStudio (Wei et al., 2017). No two environmental variables exceeded a correlation coefficient of 0.5, suggesting multicollinearity was not significant. Abundance estimates were scaled by a factor of eight (smallest factor possible) and rounded to whole integers for modeling purposes. For each GAM, logarithmic links with cubic regression splines were fitted, in order to increase the smoothness of the curve. Both models employed four degrees of freedom, to reduce the likelihood of overfitting the model, as well as creating unrealistic ecological predictions based on the limited explanatory variables (Lehmann et al., 2002; Mohan et al., 2017). A negative binomial distribution was used, due to a high abundance of zeros in the data set (Drexler and Ainsworth, 2013). GAM response plots were generated for significant environmental variables (bottom temperature and depth), whereas nonsignificant variables (salinity) were not investigated further (Table 1). In order to examine the overall fit of both generated models, percent deviance explained (DE) was calculated using the following equation and reported (Table 1):

Table 1
Temporal Variations
In addition to the generated GAMs for both white and red hake, a mapped time series of trawl data over the entire survey region was conducted. Trends in white hake abundance, red hake abundance, and bottom temperature were visualized over the past 22 years to examine spatial and temporal variability in this survey. Maps were created using ODV (version 5.5.2) using weighted average gridding. In aims to reduce outliers and display data trends, the 22-year sampling period was split into three sampling intervals of seven to eight years: (2000–2006, 2007–2014, and 2015–2021). Depth data was pooled across the 22-year sampling period (Fig. 1) and mapped using the same procedure.
Results
Overall, 1590 (x -Year±SD = 82.63 ± 24.35) individual trawls spanning from the fall of 2000 through the fall of 2021 were analyzed (Table 1). In total, 57 555 white hake were caught (x~±SD= 19.24 ± 21.76 per tow), and 77 029 red hake were caught (x~±SD= 40.36 ± 38.89 per tow) over the 22-year sampling period (Table 2). Length frequency data collected suggests the majority of the catch were small individuals (≤30 cm, white hake = 86%, red hake = 78%. Fig. 2). Distribution of length frequencies were consistent over the three sampling intervals. Environmental conditions were measured for all analyzed trawls, covering a wide range of temperature (3.6–16.6°C), salinity (26.0–34.8 ppt), and depth (6.9–223.1 m).
Table 2
Abundance Trends
Results indicate similar trends between white and red hake catch over the past 22 years (Fig. 3), with relatively low catch in the first few years of sampling (2000 – 2005). This trend changes after 2005, with both species displaying an increase in catch, with white hake hitting a maximum in 2008, and red hake hitting a maximum in 2010, with both species returning to lower abundance until around 2013. Around this time, both species begin to diverge in trends, with white hake displaying volatile abundance (sharp increase and decrease in abundance), while red hake have displayed an almost uninterrupted growth. Since 2017, white hake have shown a continuous decline in catch for this survey, the longest decline observed in the survey’s 22-year history.
Generalized Additive Models
GAMs were generated for both white and red hake, each with a deviance explained of 11.7% and 31.5% respectively. For both models, salinity was found to be an insignificant variable in abundance calculation, therefore was excluded from each model and not plotted. Both bottom temperature and depth were both found to be significant and these variables were included in each model. From the generated GAM response plots, we observe that white and red hake display distinct variations in abundance based on the environmental variables (bottom temperature and depth).
Fig. 2
Bottom Temperature
For white hake, bottom temperatures ranging from ~9 to ~13℃ displayed a positive influence on abundance. Temperatures outside that range showed a negative correlation to abundance, with warmer temperatures (> ~13℃ ) showing a considerable decrease in abundance. Of the total 57 555 white hake caught, 89% (51 402 white hake) were caught within this positive temperature window. Red hake showed a variation of this trend, where warmer temperatures (> ~12℃) showed a negative effect on abundance, with all temperatures colder (<~12℃) displaying an increased positive trend (Fig. 4). Of the total 77 029 red hake caught, 96% (73 975 red hake) were caught within this positive temperature window.
Fig. 3
Depth
For white hake, abundance showed positive correlation to a distinct range in depth (~55 to ~110 m). Depths outside this range display a negative trend in abundance, however, deeper waters ( > ~200 m) also display a positive trend in abundance. Of the total 57 555 white hake caught, 60% (34 567 white hake) were caught within this positive depth window. Red hake displayed a wide range depth where abundance was positively influenced in deeper waters (> ~65 m, Fig. 4). Of the total 77 029 red hake caught, 93% (71 451 red hake) were caught within this positive depth window. We observe that 74% of the white hake caught were in waters <100 m, while 64% of red hake were caught in waters >100 m.
Temporal Variations
A time series of spatial distribution of fish density for both white hake and red hake as well as bottom temperature for three sampling intervals (2000–2006, 2007–2014 and 2015–2021) over the 22-year sampling period was conducted (Fig. 5). As previously stated, the sampling of waters >100 m started in 2004, which is observed in our sample distribution, where tows >100 m made up 18% in the first interval (2000–2006), compared to 28% (2007–2014) and 27% (2015–2021) in the following intervals respectively. These time series showed changes in both bottom temperatures observed, as well as in hake abundance over the sampling intervals.
Fig. 4
White Hake
White hake catch show northern trends in abundance, in this spatially limited survey. Between the first (2000–2006, Fig. 5A) and second (2007–2014, Fig. 5B) sampling intervals, there are no considerable differences observed. A major hotspot in the mid sampling regions slightly intensifies and expands between periods, as well as a slight uptick in catch in the northern regions. A major change occurs between the second (2007–2014, Fig. 5B) and third (2015–2021, Fig. 5C) sampling intervals, where there is a rapid density change in northern waters. Major aggregation sites, once located in the mid sampling region, became more prominent in northern areas, and spread across the region, opposed to the once highly concentrated areas. It also appears that there is a wide depth gradient preference in this last sampling interval, with white hake being caught in all depth strata (Fig. 5C).
Red Hake
Inshore red hake abundance showed a clear increase, in a spatially limited survey. Between the first (2000–2006, Fig. 5D) and second (2007–2014, Fig. 5E) sampling intervals, there is a clear trend of aggregation sites emerging in the mid to southern deeper inshore regions, with areas surrounding also showing increased abundance. The northern most aggregation site in the second sampling interval (Fig. 5E) was also prevalent during the first sampling interval (Fig. 5F), but of less significance. A continued change occurs between the second (2007–2014, Fig. 5E) and third (2015–2021, Fig. 5F) sampling intervals, with extended aggregation sites in the deep inshore regions of the survey. Red hake abundance appears to spread along this deep inshore area, with aggregations extending both north and south.
Fig. 5
Bottom Temperature
Over the 22-year sampling period, there is a distinct bottom temperature warming trend displayed in the inshore sampling region. Between the first (2000–2006, Fig. 5G) , second (2007–2014, Fig. 5H), and third (2015–2021, Fig. 5I) sampling intervals, bottom temperatures display an increase, with warming of northern deep water regions as well. Only the southern deep water sampling region displayed a consistent cool bottom temperature of the entire sampling period. Over the three sampling intervals, we observe a clear increase in stratification of bottom temperature between areas, with less uniformity throughout the inshore environment.
Discussion
Based on our analysis, white and red hake display distinct environmental preferences for bottom temperature and depth, with no significant response to bottom salinity. Spatial and temporal trends in hake abundance and bottom temperature show correlations between northern, inshore deep water density increases in abundance, paired with inshore shallow water warming. Both species display different responses to a warming inshore environment, with white hake displaying a narrow range in preferred bottom temperature and depth, while red hake displaying a single threshold value for both environmental conditions. This highlights distinct behavioral responses to change can occur between two closely related species in the same region.
Environmental Preferences
Both white and red hake show a strong preference towards cold waters (<~12℃), with both species abundances displaying a negative relationship with warmer waters (>~13℃). White hake display a temperature corridor, (~9 to ~13℃) a characteristic not displayed by red hake. Both species displayed a distinct preference for deeper waters (> 65 m), with red hake having an extended depth range into deeper areas. Due to the nature of this survey, adults and juveniles of both sexes were all sampled for both species, with the assumption that their habitat selection is consistent in this survey region, regardless of sex or age. Due to the lack of extensive sampling of all age classes for both species, a pooled approach was deemed appropriate for this analysis. The majority of the hake sampled were small individuals (≤30 cm, white hake = 86%, red hake = 78%, Fig. 2), which may overshadow environmental preferences of the larger fish sampled. Also as previously mentioned, the sampling of waters >100 m did not begin until the fall of 2004, potentially influencing these results, especially for depth.
White Hake
In a species overview by Chang et al. (1999), juvenile white hake showed a preference of 7–16℃ in the fall survey months, at depths <75 m. These results are similar to previous studies, which report a preference for cooler waters (4–12°C) (Musick, 1974; Markle et al., 1982). Our model demonstrates a species more tolerant of warmer waters (~9–12℃ ), similar to that of Han and Kulka (2009) (4–7℃), who described white hake aggregations in the warmer southwest waters of the Grand Banks. These results indicate that white hake have both a distinct and narrow temperature preference, which may cause concern in a warming ecosystem.
Chang et al. (1999) reports age class separation across a depth gradient, with older fish found in deeper waters offshore. Since our study was conducted in inshore waters, and primarily sampled smaller fish (≤30 cm, 86%, Fig. 2) the observed behaviors/preferences described here are limited to specific size classes. This explains the preferred depth range in the generated GAM model, displaying the depth preferences of the juveniles/developing adults. The preferences of larger, adult white hake were likely overshadowed in this study, with future research focusing on their offshore habits needed.
Red Hake
As reported in Fahay (1999), juvenile and adult red hake are most prominently found in temperatures ranging from 3–16℃. The results of this study show red hake prefer colder waters (< ~12℃), with a strong negative correlation to warmer waters. This is similar to the findings of Fahay (1999), however our results suggest a lack of tolerance for warmer waters which has not been previously described. Similarly, Fahay (1999) found juvenile red hake most prevalent in shallower waters (<120 m) and adults even deeper (<300 m), which overlaps with our findings (~65 m–220 m). We found that 64% of the red hake landed were in waters >100 m, with a majority of these individuals being smaller fish (≤30 cm, 78%, Fig. 2). This is similar to the white hake results, where the preferences of juveniles were shallower waters (<120 m) and deeper waters for adults (120 m–220 m), with red hake showing a wider range offshore during their juvenile life stage compared to white hake. This extended range may allow these developing red hake to escape the pressures of a warming inshore environment, a behavior white hake are not displaying.
Habitat Selectivity
In addition to the habitat preferences of white and red hake being displayed in our analysis, we must also consider the reasoning behind their choice of residency. A major component of a species habitat selectivity is prey availability, competition, and predator avoidance (Kotler and Holt, 1989; Hugie and Dill ,1994). The diet of juveniles of both species mostly consists of polychaetes, copepods, shrimps, and other crustaceans, with adult diets consisting mainly of a variety of fish species and squids (Langton and Bowman, 1980; Steiner et al., 1982; Garman, 1983; Bowman and Michaels, 1984). Both species as juveniles have many predators as well, including larger hake, other gadoid fishes, elasmobranchs, sea birds, and pinnipeds. The habitat selectivity of these individuals is hypothesized to be a combination of factors (prey availability, predators, environmental conditions), with this study focusing on portion of that specific habitat niche (environmental conditions: bottom temperature and depth). Identifying these other factors is key, as they have the potential to better describe the habitat preferences of these species compared to environmental conditions.
Developing juvenile white hake prefer inshore eelgrass beds (Sosebee et al., 1998) but have shown the ability to settle in deeper waters (70–80 m) on the Grand Bank (Han and Kulka, 2009). Juvenile red hake prefer sea scallop beds (Markle et al., 1982; Steiner et al., 1982; Haedrich, 2003), with both species moving away from eelgrass beds but still occupying shallower waters as they mature. While we do not observe our samples to be of developing hakes, it is still noteworthy that developing individuals can tolerate shallow, warm waters during development. Han and Kulka (2009) suggests that white hake recruitment on the Grand Bank is highly impacted by environmental conditions, specifically near surface currents impacting settlement rate. These factors were not investigated in this study but may explain density changes in species distribution over the study period.
Additionally, both species have been shown to have strong preferences to fine grain, muddy substrates (Chang, et al., 1999; Fahay, 1999), all of which are details that were not investigated in this study. Similar to conclusions made by Kleisner et al. (2017), accurately predicting a species future habitat requires complex knowledge that is currently unknown for our study species. Extended research incorporating additional environmental and biological factors would provide stronger habitat predictions.
Temporal Variations
Over the 22-year sampling period, the data collected displays distinct variations in white and red hake abundance and habitat selectivity, as well as warming trends in bottom temperature. Based on the GAM model results, we conclude that there is overlap between the habitat selectivity trends of these species over time with the warming of the inshore waters, with the caveat that other unmeasured factors are also in play.
White Hake
The temporal trends in white hake abundance over the 22-year sampling period supports our hypothesis regarding the species-specific response to a warming inshore region. White hake display a northern movement in abundance, as well as an uptick in aggregations in later years, despite a decrease in abundance. These results align with findings of Hare et al. (2012) and Adams et al. (2018), which suggest that a combination of fishing pressures and a warming environment contribute to white hake distribution variations. As described in Pershing (2015), northern migrations of cool-water dependent Atlantic cod as a result of a warming GoM directly corresponded to the collapse of the once prominent fishery. This northern movement of multiple GoM species has been described as a result of a shifting Gulf Stream and weakening Atlantic meridional overturning circulation (AMOC), with risks of permanent changes in local species distributions (Mountain and Kane, 2009; Nye et al., 2011).
Red Hake
Unlike white hake, red hake did not exhibit a distinct northern movement as bottom temperatures increase, but rather a movement towards deeper inshore areas. Similar to white hake, red hake have displayed a preference for deeper waters (up to 220 m) and cooler temperatures (up to 16.6°C), making their lack of distinct northern movement highly species specific. While it is theorized that species that fall within similar taxonomic groups respond to climate pressures similarly, species specific habitat preferences may affect the way a species behaves to a changing climate (Pinsky et al., 2013). A study by Kleisner et al. (2016) described white hake as higher trophic level species compared to red hake, with a broader offshore range. This distinction was theorized to drive distribution shifts in both latitude and depth (Kleisner et al., 2016). While white hake may have the ability to thrive in northern regions due to a combination of desirable environmental variables, red hake may be able to thrive in more offshore regions instead, without the necessity of moving poleward.
Bottom Temperature
As previously suggested by Pershing (2015), a poleward shifting Gulf Stream and weakening AMOC is theorized to be the main contributor to warming in the GoM, impacting the behavior of species within the region. The results of this study are consistent with previous research, which expects bottom temperatures in the region to increase through time, especially in fall months (Pershing et al., 2015, 2021; Thomas et al., 2017), thus reducing suitable habitat for many species (Kleisner et al., 2017). Only the deep water southern region displayed a continued cool temperature, most likely a cause of depth stratification and other external forcings (currents, bathymetry). While our time series covers an extended period of time, sampling only occurred during the fall months, thus do not provide the level of fine scale detail of a year that other studies have reported. Therefore, we can only attribute the change in bottom temperature recorded for this specific season, in the inshore survey area.
Preferences of smaller hake
Although we observe correlations in our spatial data to bottom temperature, we do acknowledge that other factors not measured in this study may also affect hake habitat preferences. Our trawl data suggest that the majority of the hake samples were smaller individuals (≤30 cm, white hake = 86%, red hake = 78%, Fig. 2), therefore we feel confident that our observed temporal variations may be aligned with recruitment success and the settling preferences of juveniles/developing adults. We observe white hake preferring northern regions, while red hake preferring deeper areas of the inshore GoM. Investigations into the historical preferences of the juveniles during times of low and high abundance would allow us to conclude if juvenile hake habitat preferences are changing or constant based on recruitment success. As the GoM continues to change, understanding the preferences of juvenile groundfish like hake is essential for future management.
Limitations and Future Study
While our findings are informative for two understudied hake species in the GoM, we do acknowledge the limitations of this study. Primarily, our data comes only from fisheries independent data, targeting only inshore waters during the fall months. While this survey has strength in determining inshore presence or absence in the fall months, it does not consider seasonal shifts in abundance across the entire GoM. We propose further study combining inshore surveys and offshore surveys (i.e. NEFSC offshore trawl survey), as well as combining fisheries independent data sources with fisheries dependent data. Furthermore, habitat preference is very complex to determine, and involves a range of variables that were not recorded for this study (substrate type, prey, predators, currents, reproduction, etc.). While we are confident in our findings that these two hake species have specific bottom temperature and depth preferences, understanding their entire scope of habitat selectivity is still widely unknown. With the lack of sampling of deeper waters (> 220 m), the trend in both white and red hake abundance in deeper waters is unclear based on these models. While depth in the GoM is mostly constant, the impending threat of temperature shifts due to climate change causes greatest concern to both of these cold-water hake species. Future research using data from the NEFSC offshore trawl survey should be conducted to investigate deeper habitats in the GoM.
Conclusion
While our study is the first investigation into the direct effects of climate change on inshore white and red hake populations, there is still much unknown. The scope of sampling gives us a limited understanding regarding the pressures both species are experiencing as a result of a warming environment. While these two species share many physical and morphological characteristics, their habitat preferences and tolerance are very dissimilar. These preferences go as far as each species displaying a unique response to their changing environment. While this study does not consider all drivers of habitat preferences, the observed patterns of both species through time is hypothesized to help better inform management practices as well as highlight the gap in published data regarding these species, specifically in inshore areas. Specifically in Canadian waters, environmental aspects are considered for stock assessments (Kulka et al., 2022), a practice we suggest implementing in the GoM for hake. Ultimately, unlike the findings of Pershing et al. (2015), we do not feel that there is currently enough data analyzed to strongly support a proper mitigation plan with both white and red hake, although we feel confident that increased investigations into their life history will allow managers the ability to impose future management decisions with confidence.
Acknowledgments
This project was prepared by Benjamin R. LaFreniere under award number NA22NMF4540361 from the National Oceanic and Atmospheric Administration, U.S. Department Of Commerce. The statements, findings, conclusions, and recommendations are those of the author(s) and do not necessarily reflect the views of the National Oceanic and Atmospheric Administration or The Department of Commerce.
References
Adams, C. F., Alade, L. A., Legault, C. M. , O’Brien, L., Palmer, M. C., Sosebee, K. A., and Traver M. L. 2018. Relative importance of population size, fishing pressure and temperature on the spatial distribution of nine Northwest Atlantic groundfish stocks. PLoS One, 13. https://doi.org/10.1371/journal.pone.0196583
Ames, E. P. 2012. White hake (Urophycis tenuis) in the Gulf of Maine: Population structure insights from the 1920s. Fish. Res. 114: 56–65 https://doi.org/10.1016/j.fishres.2011.08.007
Bowman, R., and Michaels, W. 1984. Food of seventeen species of northwest Atlantic fish. NOAA Tech Mem NMFS-F/NEC-28.
Chang, S., Morse, W. W., and Berrien, P. L. 1999. White Hake, Urophycis tenuis, Life History and Habitat Characteristics. NOAA Technical Memorandum NMFS.
Cheung, W. W. L., Pauly, D., and Sarmiento, J. L. 2013. How to make progress in projecting climate change impacts. ICES Journal of Marine Science, 70: 1069–1074. https://doi.org/10.1093/icesjms/fst133
Fahay, M. P. (1999) Essential fish habitat source document: red hake, Urophycis chuss, life history and habitat characteristics. DIANE Publishing, 133 p.
Fahay, M. P., and Able, K. W. 1989. White hake, Urophycis tenuis, in the Gulf of Maine: spawning seasonality, habitat use, and growth in young of the year and relationships to the Scotian Shelf population. Can. J. Zool., 67: 1715–1724 https://doi.org/10.1139/z89-245
Fogarty, M., Incze, L., Hayhoe, K., Mountain, D., and Manning, J. 2008. Potential climate change impacts on Atlantic cod (em>Gadus morhua) off the northeastern USA. Mitig Adapt Strateg Glob. Chang. 13: 453–466. https://doi.org/10.1007/s11027-007-9131-4
Fritz. 1965. Autumn Distribution of Groundfish Species in the Gulf of Maine and Adjacent Waters, 1955–1961. American Geographical Society, 22p.
Gaichas, S. K., Link, J. S, and Hare, J. A. 2014. A risk-based approach to evaluating northeast US fish community vulnerability to climate change. ICES Journal of Marine Science, 71: 2323–2342. https://doi.org/10.1093/icesjms/fsu048
Gannon, D. P., Craddock, J. E., and Read, A. J. 1998. Autumn food habits of harbor porpoises, Phocoena phocoena, in the Gulf of Maine. Fishery Bulletin, 96: 428–437.
Garman, G. C. 1983. Observations on Juvenile Red Hake Associated with Sea Scallops in Frenchman Bay, Maine. Trans. Am. Fish. Soc., 112: 2323–2342 https://doi.org/10.1577/1548-8659(1983)112%3C212:OOJRHA%3E2.0.CO;2
Goode, A. G., Brady, D. C., Steneck, R. S., and Wahle, R. A 2019. The brighter side of climate change: How local oceanography amplified a lobster boom in the Gulf of Maine. Glob. Chang. Biol., 25: 3571–3994. https://doi.org/10.1111/gcb.14778
Haedrich, R. L. 2003. Bigelow and Schroeder’s Fishes of the Gulf of Maine. Copeia, 2003(2): 422–423. https://doi.org/10.1643/0045-8511(2003)003[0422:BR]2.0.CO;2
Han, G., and Kulka, D. W. 2009. Dispersion of eggs, larvae and pelagic juveniles of White Hake (Urophycis tenuis) in relation to ocean currents of the Grand Bank: A modelling approach. Journal of Northwest Atlantic Fishery Science, 41: 183–196 https://doi.org/10.2960/J.v41.m627
Hare, J. A., Morrison, W. E., Nelson, M. W, Stachura, M. M., Teeters, E. J., Griffis, R. B., Alexander, M. A., Scott, J. D., Alade, L., Bell, R. J., Chute, A. S., Curti, K. L., Curtis, T. H., Kircheis, D., Kocik, J. F., Lucey, S. M., McCandless, C. T., Milke, L. M., Richardson, D. E., Robillard, E., Walsh, H. J., McManus, M. C., Marancik, K. E., and Griswold, C. A. 2016. A vulnerability assessment of fish and invertebrates to climate change on the northeast U.S continental shelf. PLoS One, 11. https://doi.org/10.1371/journal.pone.0146756
Hastie, T., and Tibshirani, R. 1987. Generalized additive models: Some applications. J. Am. Stat. Assoc., 82: 371–386. https://doi.org/10.1080/01621459.1987.10478440
Hugie, D. M., and Dill, L. M. 1994. Fish and game: a game theoretic approach to habitat selection by predators and prey. J. Fish. Biol., 45: 151–169. https://doi.org/10.1111/j.1095-8649.1994.tb01090.x
Kleisner, K. M., Fogarty, M. J., McGee, S., Barnett, A., Fratantoni P., Greene J., Hare, J. A., Lucey, S. M., McGuire, C., Odell, J., Saba, V. S., Smith, L., Weaver, K. J., and Pinsky, M. L. 2016. The effects of sub-regional climate velocity on the distribution and spatial extent of marine species assemblages. PLoS One, 11. https://doi.org/10.1016/j.pocean.2017.04.001
Kleisner, K. M., Fogarty, M. J., McGee, S., Hare, J. A., Moret, S., Perretti, C. T., and Saba, V. S. 2017. Marine species distribution shifts on the U.S. Northeast Continental Shelf under continued ocean warming. Prog. Oceanogr., 153: 24–36. https://doi.org/10.1016/j.pocean.2017.04.001
Kotler, B. P., and Holt, R. D. 1989. Predation and Competition: The Interaction of Two Types of Species Interactions. Oikos, 54: No. 2. 256–260. https://doi.org/10.2307/3565279
Kulka, D., Thompson, S., Cogliati, K., Olmstead, M., Austin, D., and Pepin, P. 2022. An Accounting of Integration of Environmental Variables in Fishery Stock Assessments in Canada. Cdn. Tech. Rep. of Fish. and Aqu. Sci. 3473. 89 p. Fisheries and Oceans Canada. https://waves-vagues.dfo-mpo.gc.ca/library-bibliotheque/41060064.pdf
Lehmann, A., Overton, J. M. C., and Leathwick, J. R. 2002.GRASP: Generalized regression analysis and spatial prediction. Ecol. Modell., 157: 189–207 https://doi.org/10.1016/S0304-3800(02)00195-3
Markle, D. F., Methven, D. A., and Coates-Markle, L. J. 1982.Aspects of spatial and temporal cooccurrence in the life history stages of the sibling hakes, Urophycis chuss (Walbaum, 1792) and Urophycis tenuis (Mitchill, 1815) (Pisces: Gadidae). Can. J. Zool., 60: 2057–2078 https://doi.org/10.1139/z82-265
Mohan, J. A., Sutton, T. T., Cook, A. B., Boswell, K. M., and David Wells, R. J. 2017. Influence of oceanographic conditions on abundance and distribution of post-larval and juvenile carangid fishes in the northern Gulf of Mexico. Fish. Oceanogr., 26: 526–541. https://doi.org/10.1111/fog.12214
Mountain, D. G., and Kane, J. 2009. Major changes in the Georges Bank ecosystem, 1980s to the 1990s. Mar. Ecol. Prog. Ser., 398: 81–91. https://doi.org/10.3354/meps08323
Moyano, M., Illing, B., Polte, P., Kotterba, P., Zablotski, Y., Gröhsler, T., Hüdepohl, P., Cooke, S. J. , and Peck, M. A. 2020. Linking individual physiological indicators to the productivity of fish populations: A case study of Atlantic herring. Ecol. Indic., 113. https://doi.org/10.1016/j.ecolind.2020.106146
Musick, J. A. 1967. Designation of the hakes, (Urophycis chuss and Urophycis tenuis) in ICNAF statistics. International Commission for the Northwest Atlantic Fisheries: 2–6.
Musick, J. A.1974. Seasonal Distribution Of Sibling Hakes, Urophycis chuss And Urophycis tenuis (Pisces, Gadidae) In: New-England. Fishery Bulletin, 72: 5 p. https://www.nafo.int/Portals/0/PDFs/icnaf/docs/1967/res-76.pdf
National Marine Fisheries Service, New England Fishery Management Council. 2021. Fisheries of the Northeastern United States; Northeast Multispecies Fishery; 2021–2023 Small-Mesh Multispecies Specifications.
New England Fishery Management Council. 2022. Northeast Multispecies Fishery Management Plan for Small Mesh Multispecies. Northeast Fisheries Science Center reference document.
Northeast Fisheries Science Center. 2013. 56th Northeast Regional Stock Assessment Workshop (56th SAW) Assessment Summary Report.
2017. Operational assessment of 19 northeast groundfish stocks, updated through 2016. Northeast Fisheries Science Center reference document 17.
2019. Operational Assessment of 14 Northeast Groundfish Stocks, Updated Through 2018. Northeast Fisheries Science Center Reference Document.
Northeast Fisheries Science Center. 2020a. Fall Management Track Assessments, 2020.
2020b. Final Report of Red Hake Stock Structure Working Group.
2021. Framework Adjustment 61 To the Northeast Multispecies Fishery Management Plan Appendix III White Hake Rebuilding Plan Analysis. Northeast Fisheries Science Center reference document.
2022 Management Track Assessment Report: White Hake.
Nye, J. A., Joyce, T. M., Kwon, Y. O., and Link, J. S. 2011. Silver hake tracks changes in Northwest Atlantic circulation. Nat. Commun., 2. https://doi.org/10.1038/ncomms1420
O’Brien, L., Burnett, J., and Mayo, R. K. 1993. Maturation of nineteen species of finfish off the northeast coast of the United States, 1985–1990. NOAA Technical Report NMFS, 113.
Overholtz, W., and Link, J. 2009. A simulation model to explore the response of the Gulf of Maine food web to large-scale environmental and ecological changes. Ecol. Modell., 220: 2491–2502. https://doi.org/10.1016/j.ecolmodel.2009.06.034
Overholtz, W. J., Hare, J. A., and Keith, C. M. 2011. Impacts of Interannual Environmental Forcing and Climate Change on the Distribution of Atlantic Mackerel on the U.S. Northeast Continental Shelf. Marine and Coastal Fisheries, 3: 219–232. https://doi.org/10.1080/19425120.2011.578485
Perry, A. L., Low, P. J., Ellis, J. R., and Reynolds, J. D. 2005.Ecology: Climate change and distribution shifts in marine fishes. Science (1979), 308: 1912–1915 https://doi.org/10.1126/science.1111322
Pershing, A. J., Alexander, M. A., Brady, D. C., Brickman, D., Curchitser, E. N., Diamond, A. W., McClenachan, L., Mills, K. E., Nichols, O. C., Pendleton, D. E., Record, N. R, Scott, J. D., Staudinger, M. D., and Wang, Y. 2021. Climate impacts on the Gulf of Maine ecosystem: A review of observed and expected changes in 2050 from rising temperatures. Elementa, 9. https://doi.org/10.1525/elementa.2020.00076
Pershing, A. J., Alexander, M. A., Hernandez, C. M., Kerr, L. A., Bris, A. L, Mills, K. E., Nye, J. A., Record, N. R., Scannell H. A., Scott, J. D., Sherwood, G. D., and Thomas A. C. 2015. Slow adaptation in the face of rapid warming leads to collapse of the Gulf of Maine cod fishery. Science: 350: 809–812. https://doi.org/10.1126/science.aac9819
Pinsky, M. L., Worm, B., Fogarty, M. J, Sarmiento, J. L., and Levin, S. A. 2013. Marine taxa track local climate velocities. Science, 1979. 341. https://doi.org/10.1126/science.1239352
Richards, R. A., Fogarty, M. J., Mountain, D. G., and Taylor, M. H. 2012. Climate change and northern shrimp recruitment variability in the Gulf of Maine. Mar. Ecol. Prog. Ser., 464: 1239–1242. https://doi.org/10.3354/meps09869
Saba, V. S., Griffies, S. M., Anderson, W. G., Winton, M., Alexander, M. A., Delworth, T. L., Hare, J. A., Harrison, M. J., Rosati, A., Vecchi, G. A., and Zhang, R. 2016.Enhanced warming of the Northwest Atlantic Ocean under climate change. J. Geophys. Res. Oceans., 121. https://doi.org/10.1002/2015JC011346
Sosebee, K., O’Brien, L., and Hendrickson, L. 1998. A report of the 19th Northeast Regional Stock Assessment Workshop: a preliminary analytical assessment for white hake in the Georges Bank-Gulf of Maine region.
Steiner, W., Luczkovich, J., and Olla, B. 1982. Activity, Shelter Usage, Growth and Recruitment of Juvenile Red Hake Urophycis chuss. Mar. Ecol. Prog Ser. 7: 135–135. https://doi.org/10.3354/meps007125
Wei, T., Simko, V., Levy, M., Xie, Y., Jin, Y. J., and Zemla, J. 2017. Visualization of a Correlation Matrix. R package “corrplot”. Statistician, 56.
Citation: LaFreniere, B.R., Peters R. Donahue, B., McBride, R., and Mohan, J.A. 2023. What the Hakes? Correlating Environmental Factors with Hake Abundance in the Gulf of Maine. J. Northw. Atl. Fish. Sci., 53: 17–29. https://doi.org/10.2960/J.v54.m742