Introduction
Tanner crab (Chionoecetes bairdi) in the eastern Bering Sea are primarily distributed in Bristol Bay and the vicinity of the Pribilof Islands (Fig. 1). In this study, the Bristol Bay area is defined as east of 166.00º W, north of 54.60°N, and south of 61.85°N in the eastern Bering Sea, and the Pribilof Islands area as east of 173.00°W, west of 166.00°W, north of 54.60°N, and south of 61.85°N based on the current management areas. Somerton (MS 1981) proposed that Tanner crab in the eastern Bering Sea could be divided into two management stocks using size at maturity and estimated that at 50% maturity both male and female Tanner crab in Bristol Bay were slightly more than 10 mm larger in carapace width than those of the Pribilof Islands. Comparison of allozyme frequencies between pooled samples of 100 Tanner crab from two collections east of 162.75°W in the eastern Bering Sea and 205 Tanner crab from four collections west of 167.00°W longitude supports the division of two stocks (Merkouris et al., 1998). However, based on the summer trawl survey data of 1975–2000, Otto and Pengilly (2002) demonstrated a clinal trend in size at maturity related to depth as well as regional variation in maturity across survey years, indicating the difficulty of dividing Tanner crab in the eastern Bering Sea into two discrete stocks. Because of different sizes at maturity and genetic differences between Tanner crab in Bristol Bay and in the vicinity of the Pribilof Islands, I assumed in this study that two biologically distinct Tanner crab stocks exist in the eastern Bering Sea.
Tanner crab used to support an important fishery in the eastern Bering Sea. Tanner crab were first targeted by Japanese and Russian fleets in 1965. The fishery expanded quickly in the late 1960s with a catch of 24 000 t in 1968 (Otto, 1990). Foreign fishing for Tanner crab was reduced in the mid-1970s and has been prohibited since 1978 under the Magnuson Fisheries Conservation and Management Act. Directed fisheries for eastern Bering Sea Tanner crab by the U.S. fleet began in 1974, and catch peaked in 1978 at 31 300 t (Otto, 1990). The population collapsed in the mid-1980s, and no fishing was allowed in 1986 and 1987. The fishery was reopened in 1988, but closed during 1997–2004 because of the depressed stock condition. The fishery has been reopened for a small catch quota since 2005.
The Tanner crab fishery in the eastern Bering Sea is currently managed through a three-part harvest strategy. Firstly, the fishery is restricted by a size/sex/season approach; that is, harvest of only large (legal) males and no fishing during the spring molting and mating season. The size/sex/season approach is based on economic consideration of market value and meat yield, protection of females for reproduction, and allowance of at least one mating season for mature males. Secondly, the catch quota is based on a variable harvest rate strategy that includes a population threshold for the combined Bristol Bay and Pribilof Islands populations, variable mature male harvest rates and a maximum cap on the legal male harvest rate. Thirdly, under National Standard 1 of the Magnuson-Stevens Fishery Conservation and Management Act, the total mature biomass at maximum sustainable yield (BMSY), a minimum stock size threshold, and the fishing mortality at BMSY were established to prevent overfishing and to rebuild stocks when the stock sizes are below the minimum stock size threshold, or “overfished”.
Due to low population abundance, eastern Bering Sea Tanner crab stocks were declared “overfished” in 1999. Because total mature biomass was above the estimated BMSY for two consecutive years, the stocks reached the “rebuilt” status in 2007. However, due to changes in size at maturity and, therefore, size at terminal molt (Tamone et al., 2007), only a very small proportion of male crab can attain the legal size, and the exploitable abundance is still much lower than in the 1970s. In this study, I investigated the temporal changes in size at maturity of eastern Bering Sea Tanner crab, used abundance and environmental factors to explain the changes, and examined their implications for fisheries management.
Methods
Data
Trawl survey data for eastern Bering Sea Tanner crab from 1975 to 2006 were obtained from the U.S. National Marine Fisheries Service (NMFS). Male chela heights have been measured only since 1990. Trawl survey data include sampled crab information as well as station depth and sea surface and bottom temperatures. “Area-swept” methods (Alverson and Pereyra, 1969) were used to estimate population abundances of male crab by 5-mm width-classes and shell condition. Area-swept methods were also used to estimate female abundances by carapace width, shell condition, and maturity status. If multiple hauls were conducted for a single station during a survey, mean abundance from all hauls within the station was used. These multiple hauls were usually conducted within 2 or 3 days. Mean bottom temperatures from survey stations between 55.00°N and 58.25°N and between 160.00°W and 166.00°W during June and July each year were used as the annual temperature index for Bristol Bay, and mean bottom temperatures between 55.00°N and 58.25°N and between 166.00°W and 173.00°W in June and July each year were used for the annual index for the Pribilof Islands. Tanner crab occurred primarily within these areas. Commercial catches of eastern Bering Sea Tanner crab by width-class and shell condition were obtained from the Alaska Department of Fish and Game for the U.S. fleet from 1975 to 2006 and from Otto (1982) for the catch sampling data for the Japanese fleet from 1975 to 1977.
Classification of Maturity for Male Crab
Maturity of surveyed crab was assessed using morphometric data. For females, a prominent increase in the width of the abdomen indicates sexual maturity (Somerton, MS 1981); female maturity was visually determined during the survey. For males, morphometrically mature crab can be distinguished from morphometrically immature crab by an increase in chela height for a given carapace width (Somerton, MS 1981). Following the conventions of Conan and Comeau (1986) and Stevens et al. (1993), small-clawed crab were assumed to be morphometrically immature and large-clawed crab to be mophormetrically mature. Here, male maturity was determined using the computer program MATURE (Somerton, 1980). Three steps were used for classification of males.
First, all male carapace width and chela height data from 1990 to 2006 were combined to estimate two fitted lines for large-clawed and small-clawed crab. MATURE was used to iteratively fit two straight lines to log-transformed carapace width and chela height data: the upper line for large-clawed crab and the lower line for small-clawed crab. It started by fitting two straight lines to known small-clawed and known large-clawed crab at two ends of the data set, then extended the lines into the middle region of overlap by assigning points to the closest line, and then iteratively re-estimated the lines and reassigned data points until no points switched lines. I chose a small-clawed bound of 65 mm CW and a large-clawed bound of 150 mm CW to initialize the program. Different bounds resulted in very minor changes in classification, and these two bounds produced a relatively good fit.
Second, the average of these two lines was used as an initial dividing line between the two groups for each year. Then, this dividing line was moved up or down within the boundary of the two lines in order to find the elevation of the line that resulted in the fewest observations within its surrounding area (4.17 ≤ln(CW) ≤5.01 for the X axis and ln(ChH) ± 0.03 for the Y axis, where CW is carapace width (mm) and ChH is chela height (mm)). This adjustment of the dividing line each year was necessary because sample sizes for large-clawed and small-clawed crab differed greatly in some years. Without adjustment, the group with the larger sample size (usually large-clawed crab) would be underestimated. Finally, each data point was weighted by a scalar associated with the sub-sampling fraction from the survey, all crab were identified as “newshell” or “oldshell”, and proportions of small-claw and large-claw types within each shell condition were estimated for each 5-mm CW interval. These proportions were applied to the area-swept estimates of survey abundance. It is reasonable to believe that crab were randomly sampled for chela height measurement within a small width interval and within a shell condition but not necessary randomly sampled between shell conditions or width intervals due to likely biased sampling of large oldshell crab (R. S. Otto, NMFS, Kodiak, Alaska, personal communication).
Temporal Changes in Size at Maturity
For females, mean size is a better measure of the median size of maturity than the size at 50% maturity, because of maturing at similar ages and terminal molt at maturity (Somerton, MS 1981). Thus, annual mean sizes at maturity were estimated for female Tanner crab from 1975 to 2006. Because male Tanner crab mature at different ages and in a broad size range, sizes at 50% maturity were estimated for male Tanner crab from 1990 to 2006.
To examine variables that might influence sizes at maturity, Pearson’s product-moment correlation coefficients were calculated for mean size of mature female Tanner crab with each of the following variables: longitude and latitude of distributed centers of mature females, mean depth of mature female distribution, mature female abundance, and annual temperature index. Annual centers of distribution for mature females were calculated by averaging locations (in longitude and latitude) weighted by abundance. The strength of possible associations was examined with one-tailed t-tests in which degrees of freedom were adjusted for autocorrelation (Pyper and Peterman, 1998). Sizes at maturity were regressed against year, mean distribution depth, and longitude and latitude of distribution centers to reveal trends and explain variations.
Yield and Biomass per Recruit Analyses
Analyses of yield per recruit and male spawning biomass per recruit were used to examine the implications of changes in size at maturity for fisheries management. The length-based models used for these analyses were similar to those reported in Zheng et al. (1998), except with terminal molt at maturity for male crab and 100% molting probability for immature males. With the stock in a steady state, yield per recruit (YPR(h)) and male spawning biomass per recruit (BPR(h)) as a function of legal harvest rate h were computed as:
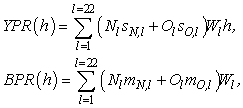 
where Nl is newshell male abundance in width-class l, Ol is oldshell male abundance in width-class l, sN,l and sO,l are the newshell and oldshell fishery selectivities, respectively, in width-class l, Wl is the mean weight in width-class l, and mN,l and mO,l are the proportions of maturity for newshell and oldshell males, respectively, in width-class l. There were 24 width classes (Table 1) with the last width class as all crab >180.5 mm. It was assumed that the fishery selectivity depended on the size limit. When the size limit changed, the width-classes that were allowed to be retained changed accordingly.
Population parameter values were updated from Zheng et al. (1998), and parameters as a function of carapace width are summarized in Table 1. Fishery selectivity was lower for oldshell crab than for newshell crab due to market preference. Natural mortality of 0.23 and 0.3 and handling mortality of 0.2 and 0.3 were used for the analyses. At time of writing, the current size limit was 138 mm CW. For simplicity, the analyses assumed that no high-grading or undersized retention occurred in the fishery; i.e., all catches above the size limit were retained, and all catches below the size limit were discarded on the grounds. A gamma distribution was used to describe growth increment variation by size with β = 1.593 (Zheng et al., 1998). Three male maturity probability functions were compared: (1) maturing at large sizes, such as in the 1970s in Bristol Bay, (2) maturing at small sizes, such as in the recent 10 years in Bristol Bay, and (3) maturing at small sizes, such as during 1990–2006 around the Pribilof Islands.
Results
Classification of Maturity for Male Crab
Log-transformed chela height and carapace width appeared as two clouds of data points (Fig. 2). The upper cloud was assumed to be morphometrically mature crab and the lower cloud to be morphometrically immature crab. There was not a clear separation of chela height between 65 and 150 mm CW (4.17 ≤ln(CW) ≤5.01). Truncation also caused the points to appear as strips for small crab. The adjusted dividing lines for individual years helped to equalize total number of points misclassified above and below the dividing lines. The dividing lines passed through the regions of least overlap between the two clouds of data points, indicating that the lines effectively divided the large majority of morphometrically immature and mature crab and that misclassification errors were likely to be symmetric.
Temporal Changes in Size at Maturity
Mean sizes of mature females have decreased with statistical significance (p <0.01) over time for both areas since 1975 (Fig. 3). Within the time series period, the decrease in mean sizes of mature females appears to have started in the late 1980s for Bristol Bay Tanner crab whereas for Pribilof Islands Tanner crab the decline was gradual and more evident during the 2000s. Mean sizes at 50% maturity for male Tanner crab in Bristol Bay in recent years have been more than 20 mm CW smaller than those observed in the early 1990s (Fig. 3); the decrease over time was statistically significant (p <0.001). Sizes at 50% maturity for male Tanner crab around the Pribilof Islands did not exhibit a downward trend during 1990–2006. Sizes at 50% maturity for males in both areas before 1990 could have been much larger than those during 1990–2006 based on survey size frequency data of male crab, but the annual chela height data were not available to estimate male maturity during the earlier period.
Spatial distributions of Bristol Bay female Tanner crab affected the temporal trends of mean sizes at maturity. Mean sizes at maturity for females east of 163.00°W did not show a temporal trend during 1975–2006, whereas the downward trend of sizes at maturity was very strong for females west of 163.00°W (Fig. 4). Because mean sizes at maturity were larger for females east of 163.00°W than west of 163.00°W (Fig. 4), changes in spatial distributions would affect mean sizes at maturity for Bristol Bay female Tanner crab. Due to a relatively small proportion of Bristol Bay mature female Tanner crab east of 163.00°W (0.211 during 1975–2006), the temporal trend of female sizes at maturity was caused by crab west of 163.00°W.
Proportions of large (>112 mm CW) males that were of commercially legal size (>137 mm CW) also indirectly indicated changes in size at maturity for male Tanner crab since 1975. Although harvest rates were much higher during the mid- and late 1970s than during the recent 10 years, there were a lot more legal males in the mature population during the earlier period than in the recent 10 years (Fig. 5). A larger relative decline was observed for Pribilof Islands Tanner crab than for Bristol Bay Tanner crab: these proportions in the Pribilof Islands area were close to 60% during the mid- and late 1970s and were about 10% during the last 10 years (Fig. 5). In contrast, Bristol Bay experienced less than a 50% decline: from approximately 50% to about 30%. The cyclic variation of these proportions was primarily caused by recruitment variation and exploitation. Strong recruitment increased legal male abundance that was in turn subjected to heavy fishing, and sublegal males suffered much less fishing mortality than legal males. When recruitment was poor, for a time period after a period of strong recruitment, these proportions were much lower than during the strong recruitment period due to high sublegal male abundance.
After adjusting for temporal autocorrelation, mean sizes of mature females were significantly correlated only with mean longitude and bottom depth in Bristol Bay and only with mean summer bottom temperature around the Pribilof Islands (Table 2). Population abundance seems statistically unrelated to sizes at maturity for Tanner crab in both areas. Bottom depth was strongly correlated with longitude and latitude in Bristol Bay; shallow waters are in the northeast and deep waters are in the southwest (Fig. 1). In Bristol Bay, mean sizes of mature females decreased from east to west and from shallow waters to deep waters (Fig. 6).
Yield and Biomass per Recruit Analyses
Maturity probability greatly affected yield and male spawning biomass per recruit. The low maturity probability for males (maturing at a large size) resulted in much higher yield per recruit than the high maturity probability (maturing at a small size) in Bristol Bay, and only a very low yield could be taken with the high maturity probability observed around the Pribilof Islands during 1990–2006 (Fig. 7). For all three levels of male maturity probabilities, yields per recruit were maximized at very high legal harvest rates, close to 0.9. As expected, mature male spawning biomass per recruit decreased as legal harvest rate increased (Fig. 7). However, the decrease in spawning biomass as a function of legal harvest rate was much less for the two levels of high maturity probability than for the lowest maturity probability. Harvest rates had limited impacts on male spawning biomass per recruit because few males grew to legal size with high maturity probabilities (Fig. 7). The reference legal harvest rate, H35%, corresponding to the F35% fishing mortality proposed as the overfishing level by the North Pacific Fishery Management Council (NPFMC, 2007), varied greatly depending on the male maturity probability. H35% was 0.64 for the low maturity probability and could not be estimated for the other two levels of high maturity probabilities because B35% did not exist for these two levels of maturity probabilities (Fig. 7).
Male maturity probability also affected predictions of yield and mature male biomass per recruit as a function of size limit (Fig. 8). At the lowest maturity probability, lowering the size limit had relatively little impact on yield and mature male biomass per recruit when legal harvest rates were low and moderate (<0.3). At the maturity probability of Pribilof Islands Tanner crab during 1990–2006, yield per recruit increased greatly at lowered size limits. As expected, high natural mortality and handling mortality rates resulted in a low predicted yield and spawning biomass per recruit (Fig. 9). Under a given legal harvest rate, benefits of lowering the size limit were greater when natural mortality and handling mortalities were high than with low mortalities (Figs. 8 and 9). Lowering the size limit and legal harvest rate simultaneously, when male crab mature at a small size, should greatly increase yield per recruit while maintaining or increasing male spawning biomass per recruit.
Discussion
Changes in size and age at maturity often occur in many fish stocks. After the population declined by 75–80% from the 1961–1966 virgin population to the exploited population of 1993–2004, both size and age at maturity decreased for northwest Atlantic porbeagle (Lamna nasus) (Cassoff et al., 2007). For Atlantic sharpnose shark (Rhizoprionodon terraenovae), size and age at maturity decreased from 78.3–80.2 cm total length and 2.3 years in 1979–1980 to 72.6–75.8 cm total length and 1.4 years in 1998–2001 (Carlson and Baremore, 2003). Size at first maturity declined about 15% from the late 1980s to the late 1990s for northwest Atlantic spiny dogfish (Squalus acanthias), perhaps due to the reduction in mature female biomass (Sosebee, 2005). Maturity during 1960–1990 for North Sea plaice (Pleuronectes platessa) occurred at a younger age and smaller size than during 1904–1911 (Rijnsdorp, 1993). After stock collapses in the early 1990s, both size and age at maturity declined sharply for the cod (Gadus morhua) stocks in Northwest Atlantic (Saborido-Rey and Junquera, 1998; Hutchings, 2005). Decreases in size and age at maturity often occurred after the stock abundances declined sharply, so density-dependent growth and maturity were commonly used to explain the temporal changes (Helser and Almeida, 1997; Saborido-Rey and Junquera, 1998; Carlson and Baremore, 2003; Schueller and Hansen, 2005; Cassoff et al., 2007). Temporal changes in size and age at maturity may also be the product of genetic responses to exploitation (Policansky, 1993; Rijnsdorp, 1993; Hutchings, 2005).
The causes for temporal changes in size at maturity for eastern Bering Sea Tanner crab have not completely been understood. Changes in spatial distribution may explain part of the temporal changes for female Tanner crab in Bristol Bay. In Bristol Bay, female Tanner crab matured at a larger size in the northeastern area than those in the southwestern area (Otto and Pengilly, 2002), and the sea bottom depths generally increase from northeast to southwest. Therefore, bottom depth, longitude, and size at maturity for Bristol Bay female Tanner crab were strongly correlated. A temporal shift of spatial distributions from northeast to southwest would cause reduction of size at maturity over time, if depth distribution actually represents a cause for changes in size or age at maturity, and not simply a covariate. However, bottom depth, longitude, and size at maturity were not statistically correlated for female Tanner crab around the Pribilof Islands.
It is not clear why female Tanner crab matured at a larger size in shallower waters in Bristol Bay. Otto and Pengilly (2002) hypothesized that immature Tanner crab migrate from deep to shallow waters and migrate more than mature Tanner crab, and crab not maturing in deep waters continue to migrate into shallower waters and mature at an older age and larger size. They also hypothesized that Tanner crab may mature at a constant age, but grow faster and to larger size at age of maturity in some shallow waters, perhaps due to warmer or more productive conditions. Unless male Tanner crab mature over a constant range of ages, the second hypothesis does not directly apply to male Tanner crab since male crab mature in a very broad size range and must mature at different ages. Somerton (MS 1981) found regional variation of maturity size of female Tanner crab in the eastern Bering Sea to be largely explained by temperature, and Taylor et al. (1993) found that a drop in the proportion of newshell snow crab (Chionoecetes opilio) off the Newfoundland coast corresponded with a sharp drop in bottom temperature. However, summertime bottom temperature did not statistically correlate with size at maturity for female Tanner crab in Bristol Bay. The statistically significant relationship between size at maturity and bottom temperature for female Tanner crab around the Pribilof Islands was negative, conflicting with the second hypothesis. Since sizes at maturity were much larger when the temperatures were cold in the early and mid-1970s than those during the last 10 years when the temperatures were relatively high, bottom temperature may not be an important factor in explaining the temporal changes in size at maturity for eastern Bering Sea Tanner crab. Due to different temporal trends of mean sizes at maturity, another possible cause for different sizes at maturity for Bristol Bay female Tanner crab is that there are two sub-stocks in Bristol Bay, approximately separated by the 163.00°W line. East of 163.00°W, either growth rates were higher or ages at maturity were older than crab west of 163.00°W, resulting in larger sizes at maturity. Tagging or aging data are currently not available to test hypothesizes regarding variability in growth and age at maturity.
Contrary to the results for many fish stocks (Helser and Almeida, 1997; Saborido-Rey and Junquera, 1998; Carlson and Baremore, 2003; Schueller and Hansen, 2005; Cassoff et al., 2007), changes in mature female abundances could not explain the decrease in size at maturity for eastern Bering Tanner crab. The density-dependent effects on size at maturity may not be linear and may be confounded with spatial differences of size at maturity. For example, although mature female abundance was relatively low during the mid-1980s, the concentration of mature females in the northeastern area of Bristol Bay may have also resulted in large sizes at maturity.
Genetic responses to size-selective fishing may also play a role on temporal changes in size at maturity for eastern Bering Sea Tanner crab. With terminal molt at maturity and a size limit for commercial fisheries, fast-growing male crab may be harvested before having an opportunity to mate and slow-growing males may stop growing before reaching the legal size limit, thus mating with females may primarily be carried out by slow-growing males. Over time, this fishing-induced mating selection may favor the slow-growing individuals and result in small sizes at maturity. The likelihood that genetic responses to fishing have occurred depends on harvest rates as well as the number of generations over which a population has been fished (Law, 2000). Harvest rates on eastern Bering Sea legal male Tanner crab reached as high as 60% in some years before the fishery was closed in 1997 (Zheng et al., 1998). However, heavy fishing started only in the late 1960s and early 1970s, only about two generations prior to the late 1980s when sizes at maturity began to decline. It is not clear whether genetic responses to fishing could occur within such a short time period. Furthermore, several Tanner crab stocks in southeast Alaska have been more heavily fished than eastern Bering Sea Tanner crab stocks (Zheng et al., 2007), yet no such temporal changes in size at maturity have been observed. Studies are needed to examine whether there are genetic differences between fast-growing Tanner crab that mature at large sizes and slow-growing individuals that mature at small sizes.
Variation in cohort strength may also affect mean sizes at maturity over time. Sizes at maturity may vary among different segments of a given cohort or year class. It is likely that the fast-growing individuals constitute the leading edge of the size distribution of the cohort, and that they have a high molting probability and attain maturity at large body sizes. Individuals with average growth rates may make up the middle of the distribution, and the slow-growing crab may form the tail end of the distribution and mature at small body sizes. Such cohort effects on size at maturity and periodic recruitment patterns would cause quasi-cyclic variations in sizes at maturity over time.
Tanner crab appear to make growth and reproduction tradeoffs: those that grow do not reproduce, and those that reproduce do not grow (Paul and Paul, 1995). In a study of the Kodiak population, only oldshell (or very oldshell) males were found grasping multiparous females (Stevens et al., 1993). Breeding and molting seasons overlap (Paul and Paul, 1995), and softshell males cannot mate within 99 d of molting (Paul et al., 1995). With terminal molt at maturity, the tradeoff may be one way. Individuals maturing at small sizes may start to reproduce early but sacrifice growth and may never attain the commercial sizes.
Temporal changes in sizes at maturity and terminal molt at first maturity have important implications on the assessment and management of the eastern Bering Sea Tanner crab fishery. As pointed out by Conan and Comeau (1986), terminal molt has implications on legal size limit and yield optimization. Sex restriction and size limit measures have been used to manage the Tanner crab fishery. Because of terminal molt at maturity, if maturity occurs before reaching legal size, crab will never be available for harvest. Conversely, if maturity occurs after reaching legal size, crab may be harvested before having an opportunity to mate, thus reducing reproductive potential and perhaps future recruitment. In terms of yield per recruit, a size limit is not an efficient management measure to optimize harvest of a cohort of crab that stop growing over a wide range of sizes. Consequently, a conflict exists between management objectives for maximizing yield or economic value per recruit and for adequately protecting stock reproductive potential. Additionally, over the long term, use of a large size limit on an exploited stock exhibiting terminal molt will result in harvesting the fastest growing segment of the stock with potential genetic consequences (Kruse, 1993; Sainte-Marie et al., 1995; Law, 2000). Presence of terminal molt also affects the choice of suitable methods to estimate growth and natural mortality, which are important population parameters used to assess stock sizes and to evaluate an optimal harvest strategy.
Modeling indicates that decrease in size at maturity will result in fewer male Tanner crab in the eastern Bering Sea to be able to grow above the current legal size limit. The current size limit was established in 1976 for two purposes: (1) preserving a brood stock by allowing males to remain at liberty for a least one year after reaching maturity and (2) reducing wastage by restricting the harvest to only those large male crab considered acceptable to processors (Somerton, MS 1981). The size limit was developed with an assumption that male Tanner crab could continue to molt after reaching maturity (Somerton, MS 1981). Based on recent years of survey length frequency data and recent studies (e.g., Tamone et al., 2007), this assumption is wrong. However, estimated molting probabilities for male crab were very high during the 1970s (Zheng et al., 1998). Although estimated median size at maturity (115 mm CW for Bristol Bay male Tanner crab during 1979–1980; Somerton, 1980) was about one molt increment smaller than the size limit, male crab largely grew above the legal size limit. chela height data were not available to estimate sizes at maturity for male Tanner crab in the eastern Bering Sea before 1979. With terminal molt at maturity, perhaps the median sizes at male maturity before 1979 were larger than that estimated for 1979–1980. With a decrease in size at maturity, few male crab could attain the legal size during recent years. Although the current size limit and decrease in size at maturity may provide added reproductive safeguards at low stock sizes, management options, such as lowering the current legal size limit, using a limit ratio of chela height against carapace width, or implementing both a maximum size limit and a minimum size limit like a slot fishery may need to be considered and evaluated to reduce the loss of harvest opportunity for small-size, mature crab and to avoid potential, adverse genetic consequences.
With crab maturing at small sizes and terminal molt at maturity, lowering the legal size limit would greatly increase yield. Because of different sizes at maturity for Bristol Bay and Pribilof Islands Tanner crab, different size limits may be needed for these two areas. Even though there is not a clear cut-off longitude to divide Tanner crab in the eastern Bering Sea into Bristol Bay and Pribilof Islands stocks (Otto and Pengilly, 2002), the current longitudinal dividing line (166.00°W) should serve well for the purpose of fisheries management. East of 166.00°W, Tanner crab were larger than those west of 166.00°. West of 166.00°W, Tanner crab displayed no clear geographic pattern of changing size at maturity, with respect to longitude. Following a decrease in size limits, harvest rates would also need to be reduced to preserve the spawning stocks. An economic analysis on size limit reduction will also be needed to evaluate the economic values of small-size crab, which may have changed since the legal size limit was first implemented in 1976. Overall, a comprehensive computer simulation study incorporating Tanner crab biological features and economic factors is needed to develop optimal combinations of size limits and harvest rates that optimize long-term, sustainable yields and protect the stock reproductive potential.
Acknowledgements
I thank helpful comments from Doug Woodby of the Alaska Department of Fish and Game and two anonymous reviewers on the earlier version of the manuscript. This paper is funded in part by a cooperative agreement from the National Oceanic and Atmospheric Administration. The views expressed herein are those of the author and do not necessarily reflect the views of NOAA or any of its sub-agencies. This is contribution PP-250 of the Alaska Department of Fish and Game, Commercial Fisheries Division, Juneau.
References
ALVERSON, D. L., and W. T. PEREYRA. 1969. Demersal fish in the Northeastern Pacific Ocean - an evaluation of exploratory fishing methods and analytical approaches to stock size and yield forecasts. J. Fish. Res. Board Can., 26: 1985–2001.
CARLSON, J. K., and I. E. BAREMORE. 2003. Changes in biological parameters of Atlantic sharpnose shark Rhizoprinodon terraenovae in the Gulf of Mexico: evidence for density-dependent growth and maturity? Mar. Fresh. Res., 54: 227–234. doi:10.1071/MF02153
CASSOFF, R. M., S. E. CAMPANA, and S. MYKLEVOLL. 2007. Changes in baseline growth and maturation parameters of Northwest Atlantic porbeagle, Lamna nasus, following heavy exploitation. Can. J. Fish. Aquat. Sci., 64: 19–29. doi:10.1139/F06-167
CONAN, G. Y., and M. COMEAU. 1986. Functional maturity and terminal molt of male snow crabs, Chionoecetes opilio. Can. J. Fish. Aquat. Sci., 43: 1710–1719.
HELSER, T. E., and F. P. ALMEIDA. 1997. Density-dependent growth and sexual maturity of silver hake in the north-west Atlantic. J. Fish. Bio., 51: 607–623. doi:10.1111/j.1095-8649.1997.tb01516.x
HUTCHINGS, J. A. 2005. Life history consequences of overexploitation to population recovery in Northwest Atlantic cod (Gadus morhua). Can. J. Fish. Aquat. Sci., 62: 824–832. doi:10.1139/f05-081
KRUSE, G.H. 1993. Biological perspectives on crab management in Alaska. In: Proceedings of the international symposium on management strategies for exploited fish populations. G.H. Kruse, D.M. Eggers, R.J. Marasco, C. Pautzke and T.J. Quinn II (eds.). University of Alaska Sea Grant, AKU-W 92-001, Fairbanks., p. 355–384. http://nsgl.gso.uri.edu/aku/akuw92001/akuw92001_part-3a.pdf
LAW, R. 2000. Fishing, selection, and phenotype evolution. ICES J. Mar. Sci., 57: 659–668. doi:10.1006/jmsc.2000.0731
MERKOURIS, S. E., L. S. SEEB, and M. C. MURPHY. 1998. Low levels of genetic diversity in highly exploited populations of Alaskan Tanner crabs, Chionoecetes bairdi, and Alaskan and Atlantic snow crabs, C. opilio. Fish. Bull., 96: 525–537.
NPFMC. 2007. Environmental assessment for proposed amendment 24 to the fishery management plan for Bering Sea and Aleutian Islands king and Tanner crabs to revise overfishing definitions. North Pacific Fishery Management Council, Anchorage, Alaska, 188 p.
OTTO, R. S. 1982. An overview of the eastern Bering Sea Tanner crab fisheries. In: Proceedings of the international symposium on genus Chionoecetes. Alaska Sea Grant Rep., AKU-Z-82-018, p. 83–115. http://nsgl.gso.uri.edu/aku/akuw82002/akuw82002_part3.pdf
1990. An overview of eastern Bering Sea king and Tanner crab fisheries. In: Proceedings of the international symposium on king and Tanner crabs. Alaska Sea Grant Rep., AKU-W-89-001, p. 9–26. http://nsgl.gso.uri.edu/aku/akuw89001/akuw89001_part1.pdf
OTTO, R. S., and D. PENGILLY. 2002. Spatiotemporal trends in Tanner crab (Chionoecetes bairdi) size at maturity. In: Crabs in cold water regions: biology, management, and economics. A. J. Paul, E. G. Dawe, R. Elner, G. S. Jamieson, G. H. Kruse, R. S. Otto, B. Sainte-Marie, T. C. Shirley and D. Woodby (eds.). University of Alaska Sea Grant, AKU-W-01-003, Fairbanks., p. 339–349. http://nsgl.gso.uri.edu/aku/akuw01003/AK-SG-02-01e.pdf
PAUL, A. J., and J. M. PAUL. 1995. Molting of functionally mature male Chionoecetes bairdi Rathbun (Decapoda: Majidae) and changes in carapace and chela measurements. J. Crust. Biol., 15: 686–692. doi:10.2307/1548818
PAUL, A. J., J. M. PAUL, and W. E. DONALDSON. 1995. Shell condition and breeding success in Tanner crabs. J. Crust. Biol., 15: 476–480.
POLICANSKY, D. 1993. Fishing as a cause of evolution in fishes. In: The exploitation of evolving resources. T. K. Stokes, J. M. McGlade, and R. Law (eds.). Springer-Verlag, Berlin., p. 3–18.
PYPER, B. J., and R.M. PETERMAN. 1998. Comparison of methods to account for autocorrelation in correlation analyses of fish data. Can. J. Fish. Aquat. Sci., 55: 2127–2140. doi:10.1139/cjfas-55-9-2127
RIJNSDORP, A. D. 1993. Fisheries as a large-scale experiment on life-history evolution: disentangling phenotypic and genetic effects in changes in maturation and reproduction of North Sea plaice, Pleuronectes platessa L. Oecologia, 96: 391-401. doi:10.1007/BF00317510
SABORIDO-REY, F., and S. JUNQUERA. 1998. Histological assessment of variation in sexual maturity of cod (Gadus morhua L.) at the Flemish Cap (north-west Atlantic). ICES J. Mar. Sci., 55: 515–521. doi:10.1006/jmsc.1997.0344
SAINTE-MARIE, B., S. RAYMOND, and J.-C. BRÊTHES. 1995. Growth and maturation of the benthic stages of male snow crab, Chionoecetes opilio (Brachyura: Majidae). Can. J. Fish. Aquat. Sci., 52: 903–924.
SCHUELLER, A. M., and M. J. HANSEN. 2005. Density-dependence of walleye maturity and fecundity in big crooked lake, Wisconsin, 1997–2003. N. Amer. J. Fish. Manag., 25: 841–847. doi:10.1577/M04-090.1
SOMERTON, D. A. 1980. A computer technique for estimating the size of sexual maturity in crabs. Can. J. Fish. Aquat. Sci., 37: 1488–1494.
MS 1981. Life history and population dynamics of two species of Tanner crab, Chionoecetes bairdi and C. opilio, in the eastern Bering Sea with implications for the management of the commercial harvest. Ph.D. dissertation, University of Washington, Seattle, 220 p.
SOSEBEE, K. A. 2005. Are density-dependent effects on elasmobranch maturity possible? J. Northw. Atl. Fish. Sci., 35: 115–124. doi:10.2960/J.v35.m492
STEVENS, B. G., W. E. DONALDSON, J. A. HAAGA, and J. E. MUNK. 1993. Morphometry and maturity of paired Tanner crabs, Chionoecetes bairdi, from shallow- and deepwater environments. Can. J. Fish. Aquat. Sci., 50: 1504–1516.
TAMONE, S. L., S. J. TAGGART, A. G. ANDREWS, J. MONDRAGON, and J. K. NIELSEN. 2007. The relationship between circulating ecdysteroids and claw allometry in male Tanner crabs: evidence for a terminal molt in the genus Chionoecetes. J. Crust. Biol., 24: 635–642. doi:10.1651/S-2802.1
TAYLOR, D. M., P. G. O’KEEFE, and C. FITZPATRICK. 1993. A snow crab, Chionoecetes opilio (Decapoda, Majidae), fishery collapse in Newfoundland. Fish. Bull., 92: 412–419.
ZHENG, J., G. H. KRUSE, and M. C. MURPHY. 1998. A length-based approach to estimate population abundance of Tanner crab, Chionoecetes bairdi, in Bristol Bay, Alaska. In: Proceedings of the North Pacific symposium on invertebrate stock assessment and management. G. S. Jamieson and A. Campbell (eds.). Can. Spec. Publ. Fish. Aquat. Sci., 125: 97–105.
ZHENG, J., J. M. RUMBLE, and G. H. BISHOP. 2007. Estimating Southeast Alaska Tanner crab abundance using pot survey and commercial catch data. Alaska Fish. Res. Bull., 12: 227–242.
|